Geological processes that have shaped Mars: Why Mars looks like it does
Article written by Jim Secosky. Jim is a retired science teacher who has used the Hubble Space Telescope, the Mars Global Surveyor, and HiRISE.
Mars looks like it does because of certain geological processes. Some of them are common to both the Earth and Mars. However, others are rare or nonexistent on the Earth. Mars shows an extremely old record of the past that is lacking on the Earth. Plate tectonics and vigorous air and water erosion has wiped out nearly all of the past geology of the Earth. In contrast, much of the Martian surface is billions of years old. Another factor that has affected the appearance of Mars is its extreme cold. The coldness of the planet makes carbon dioxide significant. It has influenced Mars both as a gas and as a solid. As a greenhouse gas, early in the history of the planet, it may have been thick enough in the atmosphere to help raise the temperature enough to permit water to flow, to carve rivers, to form lakes and an ocean. Indeed, it may have been warm enough from carbon dioxide for life to first originate on Mars and then travel to the Earth on meteorites. Today, as a solid, carbon dioxide (dry ice) produces the ubiquitous gullies found in numerous areas of the planet.
Contents
Erosion Related
As on the Earth material was laid down and then later eroded. Many spectacular scenes are present with places that were mostly eroded, but with remnants remaining in the form of buttes and mesas. Sometimes, sediments were put down in layers. As a result beautiful places were created. On the Earth we admire such layers in Monument Valley and many beautiful canyons. The same types of landscapes show up on Mars. The top layer of buttes and mesas is hard and resistant to erosion. It protects the lower layers from being eroded away. On Mars that hard, cap rock could be made from a lava flow. Many, large areas of Mars have eroded in such a fashion. The remaining structures are called mesas or buttes—if they are small in area. Some mesas and buttes show layers. Mesas show the kind of material that covered a wide area.
As on the Earth, there are landslides. However, they could be a little different since Mars has only about a third of Earth’s gravity.
Common features in certain areas of the Earth’s surface are “Yardangs.” They are found in desert areas which contain much sand. The wind blows sand and shapes the relatively soft grained deposits into the long, boat shapes of yardangs. On Mars it is thought that these forms are the result of the weathering of huge ash deposits from volcanoes. Mars has the biggest known volcanoes in the solar system. Many probably threw out much fine-grained material which was easily eroded to make vast fields of yardangs. Regions called the “Medusa Fossae Formation and Electris deposits contain thousands of yardangs.
Unlike the Earth, Mars shows landscapes that are billions of years old. In that time material has been deposited and then eroded and/or greatly changed. Some features have been “inverted.” Low areas turned into high areas. Low areas like stream beds were filled with erosion-resistant materials like lava and large rocks. Later, the surrounding, softer ground became eroded. As a result, the old stream bed now appears raised. We can tell it was originally a stream bed since the overall shape from above still looks like a stream with curves and branches.
Another structure made with erosion is a “pedestal crater.” They are abundant in regions far from the equator. These craters seem to sit on little circular shelves or pedestals.[1] In the impacting process, ejecta fell about the crater and protected the underlying ground from erosion. These craters occur where we think there was a great deal of ice in the ground. So, much of the material that disappeared was just ice. With that being said, pedestal craters give us an indication of how much ice was in the region. In some places hundreds of meters of ice-rich ground were removed to make pedestal craters.[2] [3] [4]
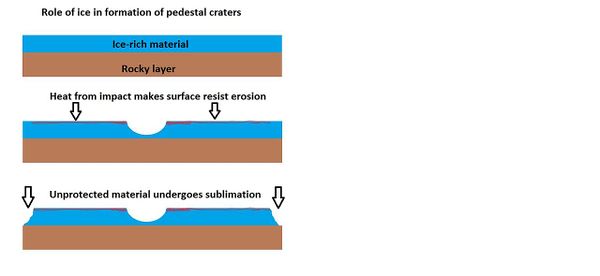
Some structures on Mars are being “exhumed.” Craters are observed that are being uncovered. In the past, impacts produced craters. Later, they were buried. Now they are in the process of being uncovered by erosion. When an asteroid strikes the surface it generates a hole and throws out ejecta all around it. A circular hole is the result. If we see a half of a crater, we know that that it is being exposed by erosion. Impacts do not produce half holes!
Craters
Impact craters occur on both the Earth and Mars. However, due to the extreme age of the Martian surface, most of Mars shows a high density of impact craters especially in the southern hemisphere. Craters do not last long on the Earth. Remember, the Earth experiences a great deal more erosion due to its thick atmosphere and abundant water. And, at intervals, the crust is taken into the Earth at plate boundaries. We know a fair amount about impact craters because the Earth has impact craters like Meteor Crater in Arizona that we can study easily.
We know that a new crater will have a rim and ejecta around it. Large ones may have a central uplift and maybe a ring around the middle of the floor. We know that the impact brings up material from deep underground.[5] If we study the rocks in the central mound and in the ejecta, we can learn about what is deep underground. During an impact, the ground is pushed down. It then rebounds and brings up rocks from deep underground.[6]
West side of Sinton Crater, as seen by CTX camera (on Mars Reconnaissance Orbiter) A central peak is visible--it occurs in larger craters and is caused by a rebound from the force of the impact.
The heat from an impact into ice-rich ground may produce channels emanating from the edge of the ejecta. These have been seen around a number of craters.
Mars shows some interesting variations to the usual appearance of craters. At times the force of an impact reaches down to a different type of layer. The lower layer may be of a different color; therefore the ejecta that is spread on the landscape may be a different color.
New, small crater Meteorite that hit here throw up dark material that was under a layer of bright, surface dust. We have found that Mars is hit by 200 impacts/year.[7]
Sometimes it looks as if an impact caused rocks to melt and when the molted rocks landed on the crater floor steam explosions occurred with ice-rich ground. What results is ground with a high density of pits.
On occasion, an impact may go down to ice-rich ground or maybe to a layer of ice. Indeed, a number of craters expose ice on their floors which after a period of time disappears into the thin Martian atmosphere.
Then there is a type of crater which is common in locations we think contain much ice. Called “ring-mold” craters, they may be caused by a rebound of an ice layer. Experiments in labs confirm that this behavior can occur. Ring-mold craters are called that because they resemble ring-molds used in baking.
Another, later idea, for their formation suggests that the impacting body goes through mantle layers of different densities. Later, erosion made the ring mold shape. The center of the crater's mantle was not eroded as much because it was compressed more due to greater depth of mantle there. It was thought that ring-mold craters could only exist in areas with large amounts of ground ice. However, with more extensive analysis of larger areas, it was found the ring mold craters are sometimes formed where there is not as much ice underground.[8] [9]
Ring-mold craters form when an impact goes through to an ice layer. The rebound forms the ring-mold shape, and then dust and debris settle on the top to insulate the ice.
In this other idea for ring mold crater formation, an impact crater and the area around it is covered with mantle layers. Next, erosion removes most of the mantle. In the crater, the central section was compressed more due to thick mantle. As a result, it became more resistant to erosion. Only the edges of the mantle were removed by erosion--a plateau results.
Now, during the impact process much material is sent flying in the air. Some of it will come down and create new craters. These are called secondary craters. They can be identified by all being of the same age. In addition, sometimes molted rock is produced by the impact. If molten rock lands on ice-rich ground, an area with a high density of pits will form. The hot molten rocks cause ice in the ground to burst into steam and cause pits to form.
Glaciers
Today, we recognize several types of features that contain ice that is covered with dirt and rocks that keep the ice from disappearing. These features behave like glaciers. Concentric crater fill (CCF), lobate debris aprons (LDA), and lineated valley fill (LVF) are their names.[10] [11] [12] [13] [14] [15] [16]
Mars may have had much water in past ages. Much of that water is now frozen in the ground and locked up in glacier-like forms. Many features have been found that are like glaciers—in that they are mostly made of ice and flow like glaciers. [17] [18] [19] [20] [21] [22] [23] That means they move slowly and in a downhill direction. For ice to exist under today’s climate conditions, it must be covered with a layer of debris—dust, rocks, etc. A layer several meters or a few tens of meters thick will preserve ice for millions of years. [24] Under today’s conditions any exposed ice would undergo sublimation and disappear into the thin Martian atmosphere. That is, it would go directly from a solid to a gas. But, the isulating effect of surface material prevents loss of ice.[21] [24] [25] [26]
Martian glaciers show evidence of movement on their surfaces and in their shapes. The actual existence of water ice in some of them has been proven with radar studies from orbit. [27] Some of them look just like alpine glaciers on the Earth. Most show piles of debris called moraine. This was material that was removed from one place and moved along to another by ice. Also, shapes looking just like eskers of terrestrial glaciers are common in places. Eskers form from streams moving under glaciers. These streams deposit rocks in tunnels in the ice at the bottom of glaciers. When the ice goes away, curved ridges stay behind.
For Mars, a number of names have been applied to these glacier-like forms. Some of them are tongue-shaped glaciers, lobate debris aprons (LDA’s), lineated valley fill (LVF), and concentric crater fill (CCF).[28] [29] [30] [31] [32] [33] [34] [35] [36] [37] [38]
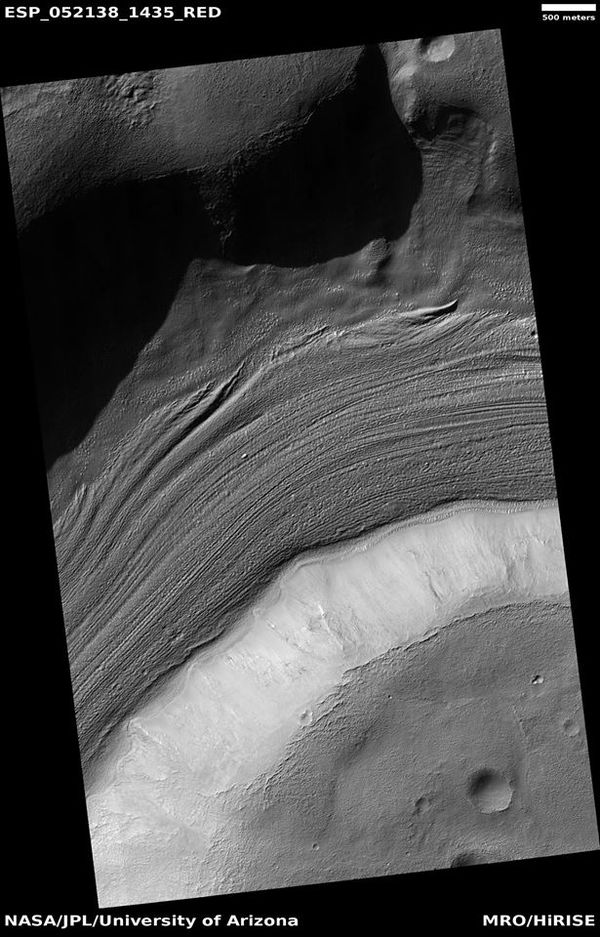
Ice in the ground
Mars has some unique landscapes and features that are common just to it. Since so much water is frozen in the ground and since the thin atmosphere of Mars allows ground ice to disappear when it became exposed, unreal scenes can develop. Under current conditions on Mars, ice sublimates when exposed to the air. In that process, ice goes directly to a gas instead of first melting. It often starts with small, narrow cracks that get larger and larger. Once ice leaves the ground there is not much left except dust. And winds will eventually carry the dust away. The end result is various shaped holes, pits, canyons, and hollows. Some of these forms are called brain terrain, ribbed terrain, hollows, scalloped terrain, and exposed ice sheets. [50] [51] All of these may be of use to future colonists who need to find supplies of water.
Open and closed brain terrain [53]
Ribbed terrain begins with cracks that eventually widen to produce hollows.
Wide view of triangular depression The colored strip shows the part of the image that can be seen in color. The wall at the top of the depression contains pure ice. This wall faces the south pole. [59]
Close view of terrain caused by ice leaving the ground Box shows size of football field.
Other signs of water ice in the ground are: lobed (rampart craters), patterned ground, and possible pingos. Pattered ground or polygonal ground is common in ice-rich areas on Earth.
Polygons Ice is in the low troughs that lie between the polygons.[64]
Pingos are mounds that contain a core of ice.[65] [66] [67] They often have cracks on their surfaces. Cracks form when water freezes and expands. Pingos would be useful as sources of water for future colonies on the planet.
Craters with ejecta that look like they were made by an impact into mud are called lobed or rampart craters. They were discovered by early, orbital missions to Mars. They are most common where we expect ice in the ground.
Channels are sometimes found in a crater's ejecta or along the edges of the ejecta. Heat from the ejecta probably melted ice in the ground. Much heat is produced with an impact.
Liquid water
Mars used to have lots of water and maybe a much thicker atmosphere billions of years ago. With liquid water, life is possible. Indeed, life may have first appeared on Mars before it occurred on Earth. Martian organisms could have been knocked off Mars by low angle asteroid impacts and found their way to Earth. Perhaps, the DNA of all Earthly organisms, included us, still contains genes from early Martian life. When we have samples of Mars brought back to Earth, we may find traces of DNA that are like ours. Data are still being gathered and ideas debated, but scientists think that once Mars cooled down and lost its magnetic field, the solar wind may have carried away much of its atmosphere. In addition, some researchers have suggested that some of the atmosphere was splashed out by impacts. After the planet cooled, water became frozen in the polar ice caps and in the ground. But, for some period there was liquid water.
Artist’s conception of how the solar wind strikes Mars, but does not reach the Earth’s surface because of the Earth’s magnetic field
This poster made by NASA shows the different ways that Mars lost most of its atmosphere after its magnetic field disappeared.
Huge amounts of water had to be present to carve the many outflow channels and produce the valley networks. Many of the outflow channels begin in "Chaos Terrain." Such a landscape often is where the ground seems to have just collapsed into giant blocks.[68] [69] It is believed that a shell of ice was created when the planet's climate cooled. Perhaps, at times the shell was broken by asteroid impacts, movements of magma, or faults. Such events would allow pressurized water to rapidly escape from under the shell of ice (shell has been called a cryosphere). Evidence is accumulating for the existence of an ocean. Lakes existed in low spots, especially craters.
Streamlined forms in wide channel
These forms were shaped by running water.
Ister Chaos Water may have come out of this landscape when the ground broke up into blocks.[70]
At present, it is hotly debated just how long water stayed around. The sun was not as strong billions of years ago. Greenhouse gases like carbon dioxide, methane, and hydrogen may have made Mars warm enough for liquid water. Massive volcanoes would have given up many of these gases, along with water vapor.
Maybe the water just existed for short periods. Some studies have showed that large impacts into icy ground could release water and change the local climate for thousands of years. Also, impacts may have punctured an ice shell and allowed pressurized water to flow out for a time. Any water moving on the surface would quickly freeze at the top. But, it would continue to flow under the ice for a long time and make many of the channels we see today. Heat to allow water to flow may have been from underground flows of magma. On the other hand, many of the features created by liquid water could have formed under massive ice sheets where water was insulated from the Martian atmosphere.
Layers
Many locations display layered formations. Some are mostly just made of ice and dust. These types of layers are common in the polar ice caps, especially the northern ice cap.[71] Other, rockier layers, are visible in the walls of impact craters and canyon walls.
And then there are layers that may be more recent, they may be connected to repeated climate changes. Some have regularity to them. The climate of Mars changes drastically due to changes in the tilt of its rotational axis. At times, like now, it is close to the Earth’s 23.5 degrees. But, at times it may be as much as 70 degrees.[72] [73] [74] Tilt governs the seasons and where ice is distributed. Currently, the largest deposit of ice is at the poles. At other times could have been at mid-latitudes. Imagine how it would be to have Pittsburgh under an ice cap. Mars may have had ice caps at the latitude of Pittsburgh.
There is an ice-rich material that falls from the sky. It is called latitude dependent mantle.[75] It is thought to come from snow and ice-coated dust. At times, there is a lot of dust in the air. When that happens, moisture will freeze onto dust grains. When the ice-coated dust particle gets heavy enough, it will fall. Recent accumulations of this mantle look smooth. In some places the mantle is layered. Some formations, particularly in protected spots in craters and against mounds, suggest that these layered formations had many more layers. The wind sometimes shapes them into layered mounds.
Mantle in a crater The mantle here has made everything look smooth on one side of the crater.
Layers in crater They were protected from erosion by being in the crater.
The older layers visible on crater and canyon walls may have different sources. Some are from lava flows or ash from volcanoes. Some may have formed under water like most layered sedimentary rocks on the Earth.[76] Curiosity, our robotic explorer, has found that layers in Gale Crater were made from sediments at the bottom of a lake. Some may be just from dust and debris settling in low areas and then being cemented by rising groundwater carrying minerals like sulfates and silica.[77] [78] [79] Sometimes a crater may have been filled up with layered rocks and then the rocks may have been eroded by the wind in such a way to just leave a layered mound in the center of the crater. Gale crater, where Curiosity is exploring, was like that.
Gale Crater with Aeolis Mons rising from the center. The noted Curiosity landing area is near Peace Vallis in Aeolis Palus. Curiosity landed in the northern part of the crater. Colors indicate elevation.
Rock layers in the Murray Buttes area in lower Mount Sharp
Igneous effects
Volcanic vent with lava channel
Igneous refers to rock that is heated to a molten condition. On Mars, this is a major shaper of landscapes. Lava comes out of the ground at holes called vents. Flows of lava can be about as fluid as water and move long distances. Sometimes the top cools to a solid, but the liquid rock continues to flow underneath a hard crust. Giant pieces of this stiff crust can move around as “lava rafts.”
In other places, lava travels in channels. When they make a hard crust, lave tunnels are created. A picture below shows lava tunnels.[80] After the liquid lava moves away, an empty tunnel can be formed. These are significant for future colonists as they may be where our first colonies will be built. There people would be protected from surface radiation. We have already found spots that might be openings to these tunnels in HiRISE images.
There are huge volcanoes that were noticed by our first spacecraft to orbit the planet. The first satellite to orbit the planet was only able to see a few volcanoes peeking above a massive global dust storm. Since Mars has not had plate tectonics for nearly all of its history, volcanoes can grow very large.[81] Lava and ash can erupt from the same spot for long periods of time. On the Earth, the plates move so volcanoes can only grow so big.
Volcanoes are only the surface manifestations of liquid rock. There is more molted rock moving under the surface than what we see above ground in volcanoes. Molted rock is called magma when underground. Stretching out around volcanoes underground are various structures. Vast linear walls, called dikes radiate out from volcanoes. On Mars they can be many miles in length. Many form by moving along cracks or weak parts of rocks. Some scientists have suggested that they from long troughs when they melt ground ice. Troughs are some of the longest features on Mars.
Besides the direct action of lava and magma, volcanoes affect Mars with just their weight. The mass of a volcano stretches the crust and makes cracks form. The large canyon system of Valles Marineris may have been started with some sort of stretching of the crust. But, its stretching may have been caused by rising mantle plumes or maybe the rise of Tharsis where so many volcanoes are located.[82] Cracks in the crust are called faults. Faults on Mars are often double faults. A center section is lower than the sides. This arrangement is called a graben. On the Earth they can turn into lakes like Lake George in New York State. Graben on Mars can be thousands of miles long.
Researchers have discovered that there is a large plume under Cerberus Fossae. It is almost the area of the continental United States. The plume is warmer than its surroundings by 95 to 285 degrees Celsius (171 to 513 degrees Fahrenheit). Plumes like this are like the plume under Hawaii.
Evidence for the plume are (1) origin of nearly all Marsquakes, (2) a rise of a mile above the surroundings, (3) crater floors tilted away from the rise, and (4) slight variations in the gravity field showing that the uplift is supported from deep within the planet. Cerberus Fossae is in Elysium Planitia, a site of the youngest known volcanic eruption on Mars. That eruption produced a small explosion of volcanic ash around 53,000 years ago--a short time in geology.
The discovery of a plume increases the chance of life. Heat from the plume could melt ground ice; consequently, allowing chemical reactions that could sustain life.[83] [84] [85]
Troughs showing layers The center section of the picture is in color. With HiRISE only a strip in the middle is in color. These troughs are in Cerberus Fossae, as seen by HiRISE under the HiWish program. Location is 15.819 N and 161.448 E. Cerberus Fossae is the source of most of the Marsqukes detected by the InSight mission.
Straight trough is a fossa that would be classified as a graben. Curved channels may have carried lava/water from the fossa. Picture taken with HiRISE under HiWish program.
Sometimes lava moves over frozen ground. That results in steam explosions. Large fields of small cones can be produced when this happens. Those cones are called “rootless cones” since they do not go down very far.
Volcanoes sometimes explode with great amounts of ash that travels long distances, covering everything. Some of the layers seen on Mars are probably from these ash deposits. These deposits do not contain boulders and are easily eroded by just the wind. Two areas on Mars have widespread and thick deposits made in this way; they are called the Medusae Fossae Formation and the Electris deposits. These relatively soft deposits often form shapes called yardangs. They are sort of boat shaped and show the direction of the prevailing wind when they were created.
Much of the atmosphere of Mars came from volcanoes. Volcanoes give off large amounts of carbon dioxide and water, along with other chemicals. Some of these chemical compounds are “greenhouse gases” that served to heat up early Mars. A few places are thought to be where volcanoes erupted under ice. The shapes that resulted look like those made on Earth when a volcano erupted under the ice.
Large group of concentric cracks Location is Ismenius Lacus quadrangle. Cracks were formed by a volcano under ice.
Bright dust
A thin coating of bright-toned dust covers almost all parts of Mars. It has a rust brown color. It is not too noticeable until it is not here. Some things remove the dust and then reveal the dark underlying surface. The contrast between this thin coating and the underlying dark rock is striking. Much of the difference derives from how NASA pictures are processed.[86] To bring out more detail, the brightest tone is considered white, while the darkest black. It only takes a very thin layer of dust to make a difference in the over-all appearance of a picture. Experiments on Earth found that the layer may be only as thick as the diameter of a human hair.[87] Incidentally, the dust has the color of rust because it is rust—it is oxidized iron.
Dark slope streaks are generally believed to occur when bright dust avalanches down steep slopes like crater walls. However, some researchers suggest that water may be involved.[88] Dust devils have been observed to start the formation of dark slope streaks.[89] [90] Streaks can be very long and elaborate. These movements are affected by obstacles like boulders. A streak may split into two when encountering a boulder. They may be initiated when an impact happens nearby.[91][92] [93]
Dark slope streaks on layered mesa
Another thing that causes light and dark patterns is a dust devil. These miniature tornadoes remove the bright dust and make straight and/or curved tracks. They are common especially in areas with much dust cover and at certain times of the day. They have been observed both from orbit and from the ground.[94] We even have movies of them in action. They can form beautiful scenes. And, the arrangement of the tracks can be different in just a few months.[95]
The atmosphere of Mars contains a great deal of fine dust. Large dust storms happen just about every Martian year. A year on Mars is about 23 of our months. Dust storms typically occur when it is spring or summer in the southern hemisphere. At that time, Mars is at its closest to the sun. Unlike the Earth, Mars has a very elliptical orbit which brings it much closer to the sun than at other times. This makes for differences in season both in intensity and length. For example the southern summer is much shorter than that of the north. However, the summer season in the southern hemisphere is much more intense.
Comparison of the orbits of Earth and Mars. The Earth’s orbit is almost a perfect circle. Mars changes its distances to sun a great deal--this changes makes drastic seasonal changes.
Dry Ice
Some of the strangest things on Mars involve dry ice—solid carbon dioxide. The cold conditions on Mars cause much of the carbon dioxide to freeze out of the atmosphere. Both ice caps contain some dry ice. Each year about 25% of the atmosphere freezes out onto the poles. This is so much that the gravity of the planet shifts. [98] [99] Winds and weather systems that almost look like the Earth’s are produced by so much dry ice changing to a gas at these times.
In the winter dry ice accumulates. So, large areas appear white. When things warm up in the spring, the landscape gets many dark spots and areas. [100][101] [102] In the past, observers thought that Mars was full of life. They saw the northern ice cap get smaller and smaller. At the same time, they watched the area get darker. They concluded that the darkening was vegetation growing from the water coming out of the ice caps. What was happening was the dry ice was disappearing. Today, we can watch this darkening occur in great detail. [103]
In some places, there are many geyser-like eruptions of gas and dark dust.[104] High pressure gas and dust explode out of the ground. Winds often blow these eruptions into dark plumes. After many observations, scientists concluded that what happens is that a transparent-translucent dry ice slab forms in the winter. With increased sun in the spring, pressure builds up under this slab as light heats cavities under the slab and causes dry ice to turn into a gas. At weak areas in the slab, the gas comes out along with dark dust.[105] [106] The channels may get dark from the dust and make a pattern that looks like a spider. These patterns are called “spiders.” [107] [108] [109] [110] [111] [112] The official name for spiders is "araneiforms."[113]
Drawing showing the cause of plumes and spiders. In the spring, sunlight goes through a clear slap of dry ice. It heats up the dark ground. Heat causes dry ice to turn into a gas and pressurize. When pressure is great enough a dark plume of carbon dioxide gas and dark dust erupt. Wind will form it into a fan shape plume.
Close view of spiders
Around the southern cap, dry ice makes round, low areas that look like Swiss cheese. [114] [115] [116] [117] So, it is called “Swiss cheese terrain.” The roundness of the pits is believed to be related to the low angle of the sun.[118]
HiRISE view of South Pole Terrain.
The ice caps contain a great deal of water ice. The northern cap has a covering of dry ice only 1 meter thick in the winter, but the southern cap always has a coating of dry ice up to 8 meters thick. Large deposits of dry ice are also buried in the water ice of the cap at some locations.
Gullies
Since 2000, researchers have been studying gullies that are common in the mid-latitudes on steep slopes. They look like they were carved by liquid water. After many years of observations, it has been concluded that today they are being made by chunks of dry ice sliding down slopes.[119] [120] [121] However, some scientists concede that water may have been involved in their formation in the past.[122] [123] [124]
Gullies near Newton Crater
Other features
The surface of Mars is very old—billions of years. This is plenty of time for rocks to have broken down into sand. In low places, like crater floors, sand accumulates and makes dunes. Some are quite pretty. And the colors used by NASA make them even more pretty—they can appear blue, purple, green, or turquoise.
Colorful dunes in the Mare Tyrrhenum quadrangle[127]
Related to dunes are something called transverse aeolian ridges (TAR’s). They look like small dunes. They are often parallel to each other. They generally are in low areas and one of the most common landforms on Mars.[128] They are mid-way in height between dunes and ripples; they are not well understood.[129] [130]
Some landscape expressions are mysteries.[131] [132]
In rocks of certain ages, often at the bottom of low spots are complex arrangements of ridges. These are walls of rock.[133]
There are different ideas for what caused them.[134] Over 14,000 people from around the world helped map them, so that scientists could better understand them. The team of volunteers found 952 polygonal ridge networks in an area that measures about a fifth of Mars’ total surface area. Some ridges contain clays, so water may have been involved in their formation because clays need water to be formed.[135] [136]
Linear ridge networks
Map of Linear ridge networks
Of eerie beauty are odd arrangements visible on the bottom of the Hellas Impact basin. We are not sure exactly what caused them. They have been called honeycomb terrain or banded terrain.
Wide view of features on floor of Hellas impact basin.
Close view of center of a Hellas floor feature
Close-up of banded terrain on the floor of the Hellas basin, as seen by HiRISE
Honeycomb terrain on floor of Hellas Basin The exact origin of these shapes is unknown at present.
Mars is one planet that we can see the surface clearly. Its super thin atmosphere (about 1% of the Earth’s) makes it easy to observe. Early telescopes revealed many markings and patterns. As we sent better and better cameras to examine it, more mysteries and more beautiful scenes emerged. We were able to answer many questions, but always more questions arose concerning what we were seeing.
References
- ↑ https://www.uahirise.org/hipod/PSP_008508_1870
- ↑ Bleacher, J. and S. Sakimoto. Pedestal Craters, A Tool For Interpreting Geological Histories and Estimating Erosion Rates. LPSC
- ↑ https://web.archive.org/web/20100118173819/http://themis.asu.edu/feature_utopiacraters
- ↑ = McCauley, John F. 1972. Mariner 9 Evidence for Wind Erosion in the Equatorial and Mid-Latitude Regions of Mars. Journal of Geophysical Research: 78, 4123–4137(JGRHomepage). |doi = 10.1029/JB078i020p04123
- ↑ https://www.uahirise.org/hipod/PSP_007464_1985
- ↑ https://www.uahirise.org/ESP_013514_1630
- ↑ https://www.space.com/21198-mars-asteroid-strikes-common.html
- ↑ https://www-sciencedirect-com.wikipedialibrary.idm.oclc.org/science/article/pii/S0019103518301532
- ↑ Baker, D. and L. Carter. 2019. Probing supraglacial debris on Mars 2: Crater morphology. Icarus. Volume 319. Pages 264-280
- ↑ https://www.sciencedirect.com.wikipedialibrary.idm.oclc.org/science/article/pii/S0019103520304619#bb0190
- ↑ Jawin, E, and J. Head. 2021. Patterns of late Amazonian deglaciation from the distribution of martian paraglacial features. Icarus. Volume 355. 114117
- ↑ S.W. Squyres. 1979. The distribution of lobate debris aprons and similar flows on Mars. J. Geophys. Res. Solid Earth, 84. pp. 8087-8096, 10.1029/JB084iB14p08087
- ↑ J.W. Head, G. Neukum, R. Jaumann, H. Hiesinger, E. Hauber, M. Carr, P. Masson, B. Foing, H. Hoffmann, M. Kreslavsky, S. Werner, S. Milkovich, S. van Gasselt, Co-Investigator Team, T.H. 2005. Tropical to mid-latitude snow and ice accumulation, flow and glaciation on Mars. Nature, 434. pp. 346-351
- ↑ J.W. Head, D.R. Marchant, J.L. Dickson, A.M. Kress, D.M. Baker. 2010. Northern mid-latitude glaciation in the late Amazonian period of Mars: criteria for the recognition of debris-covered glacier and valley glacier landsystem deposits Earth Planet. Sci. Lett., 294. pp. 306-320
- ↑ D.M.H. Baker, J.W. Head, D.R. Marchant. 2010. Flow patterns of lobate debris aprons and lineated valley fill north of Ismeniae fossae, Mars: evidence for extensive mid-latitude glaciation in the late Amazonian. Icarus, 207 (2010), pp. 186-209, 10.1016/j.icarus.2009.11.017
- ↑ J.S. Levy, J.W. Head, D.R. Marchant. 2010. Concentric crater fill in the northern mid-latitudes of Mars: formation processes and relationships to similar landforms of glacial origin. Icarus. 209. pp. 390-404, 10.1016/j.icarus.2010.03.036
- ↑ cite journal | last1 = Squyres | first1 = S.W. | last2 = Carr | first2 = M.H. | year = 1986 | title = Geomorphic evidence for the distribution of ground ice on Mars | url = | journal = Science | volume = 213 | issue = | pages = 249–253 | doi = 10.1126/science.231.4735.249 |
- ↑ cite journal | last1 = Head | first1 = J.W. | last2 = Marchant | first2 = D.R. | last3 = Dickson | first3 = J.L. | last4 = Kress | first4 = A.M. | year = 2010 | title = Criteria for the recognition of debris-covered glacier and valley glacier landsystem deposits | url = | journal = Earth Planet. Sci. Lett. | volume = 294 | issue = | pages = 306–320 | doi=10.1016/j.epsl.2009.06.041 |
- ↑ cite journal | last1 = Holt | first1 = J.W. | display-authors = 1 | last2 = et al | year = 2008 | title = Radar sounding evidence for buried glaciers in the southern mid-latitudes of Mars | url = | journal = Science | volume = 322 | issue = | pages = 1235–1238 | doi=10.1126/science.1164246 | pmid=19023078|
- ↑ | last1 = Morgan | first1 = G.A. | last2 = Head | first2 = J.W. | last3 = Marchant | first3 = D.R. | year = 2009 | title = Lineated valley fill (LVF) and lobate debris aprons (LDA) in the Deuteronilus Mensae northern dichotomy boundary region, Mars: Constraints on the extent, age and episodicity of Amazonian glacial events | url = | journal = Icarus | volume = 202 | issue = | pages = 22–38 | doi=10.1016/j.icarus.2009.02.017 |
- ↑ 21.0 21.1 cite journal | last1 = Plaut | first1 = J.J. | last2 = Safaeinili | first2 = A. | last3 = Holt | first3 = J.W. | last4 = Phillips | first4 = R.J. | last5 = Head | first5 = J.W. | last6 = Sue | first6 = R. | last7 = Putzig | first7 = A. | year = 2009 | title = Frigeri Radar evidence for ice in lobate debris aprons in the mid-northern latitudes of Mars | doi = 10.1029/2008gl036379 | journal = Geophys. Res. Lett. | volume = 36 | issue = | page = L02203 |
- ↑ cite journal | last1 = Baker | first1 = D.M.H. | last2 = Head | first2 = J.W. | last3 = Marchant | first3 = D.R. | year = 2010 | title = Flow patterns of lobate debris aprons and lineated valley fill north of Ismeniae Fossae, Mars: Evidence for extensive mid-latitude glaciation in the Late Amazonian | url = | journal = Icarus | volume = 207 | issue = | pages = 186–209 | doi=10.1016/j.icarus.2009.11.017 |
- ↑ cite journal | last1 = Arfstrom | first1 = J. | year = 2005 | title = Terrestrial analogs and interrelationships | url = | journal = Icarus | volume = 174 | issue = | pages = 321–335 | doi=10.1016/j.icarus.2004.05.026 |
- ↑ 24.0 24.1 cite journal | last1 = Williams | first1 = K. E. | display-authors = 1 | last2 = et al | year = 2008 | title = Stability of mid-latitude snowpacks on Mars | url = | journal = Icarus | volume = 196 | issue = 2| pages = 565–577 | doi=10.1016/j.icarus.2008.03.017 |
- ↑ cite journal | doi = 10.1038/nature03359 | last1 = Head | first1 = J. | date = 2005 | last2 = Neukum | first2 = G. | last3 = Jaumann | first3 = R. | last4 = Hiesinger | first4 = H. | last5 = Hauber | first5 = E. | last6 = Carr | first6 = M. | last7 = Masson | first7 = P. | last8 = Foing | first8 = B. | last9 = Hoffmann | first9 = H. | last10 = Kreslavsky | first10 = M. | last11 = Werner | first11 = S. | last12 = Milkovich | first12 = S. | last13 = Van Gasselt | first13 = S. | last14 = Co-Investigator Team | first14 = The Hrsc | title = Tropical to mid-latitude snow and ice accumulation, flow and glaciation on Mars | url = | journal=Nature | volume = 434 | issue = 7031| pages = 346–350 | pmid=15772652|
- ↑ Head, J., et al. 2009. Northern mid-latitude glaciation in the Late Amazonian period of Mars: Criteria for the recognition of debris-covered glacier and valley glacier landsystem deposits. Earth and Planetary Science Letters. Doi:10.1016/j.epsl.2009.06.041
- ↑ http://news.discovery.com/space/mars-ice-sheet-climate.html
- ↑ Levy, J., J. Head, D. Marchant. 2010. Concentric Crater fill in the northern mid-latitudes of Mars: Formation process and relationships to similar landforms of glacial origin. Icarus 2009, 390-404.
- ↑ Levy, J., J. Head, J. Dickson, C. Fassett, G. Morgan, S. Schon. 2010. Identification of gully debris flow deposits in Protonilus Mensae, Mars: Characterization of a water-bearing, energetic gully-forming process. Earth Planet. Sci. Lett. 294, 368–377.
- ↑ http://hirise.lpl.arizona.edu/ESP_032569_2225
- ↑ Garvin, J., S. Sakimoto, J. Frawley. 2003. Craters on Mars: Geometric properties from gridded MOLA topography. In: Sixth International Conference on Mars. July 20–25, 2003, Pasadena, California. Abstract 3277.
- ↑ Plaut, J. et al. 2008. Radar Evidence for Ice in Lobate Debris Aprons in the Mid-Northern Latitudes of Mars. Lunar and Planetary Science XXXIX. 2290.pdf
- ↑ http://hirise.lpl.arizona.edu/PSP_009535_2240
- ↑ Carr, M. 2006. The Surface of Mars. Cambridge University Press. ISBN|978-0-521-87201-0
- ↑ Squyres, S. 1978. Martian fretted terrain: Flow of erosional debris. Icarus: 34. 600-613.
- ↑ Levy,J. et al. 2007. Lineated valley fill and lobate debris apron stratigraphy in Nilosyrtis Mensae, Mars: Evidence for phases of glacial modification of the dichotomy boundary. J. Geophys. Res. 112
- ↑ Garvin, J. et al. 2002. Lunar Planet. Sci: 33. Abstract # 1255.
- ↑ http://hiroc.lpl.arizona.edu/images/PSP/diafotizo.php?ID=PSP_111926_2185
- ↑ Baker, D., et al. 2009. Flow patterns of lobate debris aprons and lineated valley fill north of Ismeniae Fossae, Mars: Evidence for extensive mid-latitude glaciation in the Late Amazonian. Icarus: 207. 186-209.
- ↑ Marchant, D. and J. Head. 2007. Antarctic dry valleys: Microclimate zonation, variable geomorphic processes, and implications for assessing climatic change on Mars. Icarus: 192.187-222
- ↑ Dickson, J., et al. 2008. Late Amazonian glaciation at the dichotomy boundary on Mars: Evidence for glacial thickness maxima and multiple glacial phases. Geology: 36 (5) 411-415
- ↑ Kress, A., et al. 2006. The nature of the transition from lobate debris aprons to lineated valley fill: Mamers Valles, Northern Arabia Terra-Deuteronilus Mensae region on Mars. Lunar. Planet. Sci. 37. Abstract 1323
- ↑ Levy, J., J. Head, D. Marchant. 2010. Concentric Crater fill in the northern mid-latitudes of Mars: Formation process and relationships to similar landforms of glacial origin. Icarus 2009, 390-404.
- ↑ Levy, J., J. Head, J. Dickson, C. Fassett, G. Morgan, S. Schon. 2010. Identification of gully debris flow deposits in Protonilus Mensae, Mars: Characterization of a water-bearing, energetic gully-forming process. Earth Planet. Sci. Lett. 294, 368–377.
- ↑ http://hirise.lpl.arizona.edu/ESP_032569_2225
- ↑ Garvin, J., S. Sakimoto, J. Frawley. 2003. Craters on Mars: Geometric properties from gridded MOLA topography. In: Sixth International Conference on Mars. July 20–25, 2003, Pasadena, California. Abstract 3277.
- ↑ Dickson, J., et al. 2009. Kilometer-thick ice accumulation and glaciation in the northern mid-latitudes of Mars: Evidence for crater-filling events in the Late Amazonian at the Phlegra Montes. Earth and Planetary Science Letters.
- ↑ cite web|url=http://hirise.lpl.arizona.edu/PSP_001926_2185%7Ctitle=HiRISE - Concentric Crater Fill in the Northern Plains (PSP_001926_2185)|author=|date=|website=hirise.lpl.arizona.edu
- ↑ Levy, J. et al. 2009. Concentric crater fill in Utopia Planitia: History and interaction between glacial "brain terrain" and periglacial processes. Icarus: 202. 462-476.
- ↑ Levy, J., et al. 2009. Concentric crater fill in Utopia Planitia: History and interaction between glacial "brain terrain" and periglacial mantle processes. Icarus: 202, 462-476.
- ↑ https://www.uahirise.org/hipod/PSP_001938_2265
- ↑ Levy, J., J. Head, D. Marchant. 2009. Concentric crater fill in Utopia Planitia: History and interaction between glacial “brain terrain” and periglacial mantle processes. Icarus 202, 462–476.
- ↑ Levy, J., J. Head, D. Marchant. 2009. Concentric crater fill in Utopia Planitia: History and interaction between glacial “brain terrain” and periglacial mantle processes. Icarus 202, 462–476.
- ↑ https://www.uahirise.org/hipod/PSP_001938_2265
- ↑ Lefort, A.; Russell, P. S.; Thomas, N.; McEwen, A. S.; Dundas, C. M.; Kirk, R. L. 2009. "Observations of periglacial landforms in Utopia Planitia with the High Resolution Imaging Science Experiment (HiRISE)". Journal of Geophysical Research. 114 (E4).
- ↑ Morgenstern, A; Hauber, E; Reiss, D; van Gasselt, S; Grosse, G; Schirrmeister, L (2007). "Deposition and degradation of a volatile-rich layer in Utopia Planitia, and implications for climate history on Mars" (PDF). Journal of Geophysical Research: Planets. 112 (E6): E06010.
- ↑ Zanetti, M.; Hiesinger, H.; Reiss, D.; Hauber, E.; Neukum, G. 2009. "Scalloped Depression Development on Malea Planum and the Southern Wall of the Hellas Basin, Mars" (PDF). Lunar and Planetary Science. 40. p. 2178, abstract 2178.
- ↑ Lefort, A.; Russell, P.S.; Thomas, N. (2010). "Scalloped terrains in the Peneus and Amphitrites Paterae region of Mars as observed by HiRISE". Icarus. 205 (1): 259.
- ↑ Supplementary Materials Exposed subsurface ice sheets in the Martian mid-latitudes Colin M. Dundas, Ali M. Bramson, Lujendra Ojha, James J. Wray, Michael T. Mellon, Shane Byrne, Alfred S. McEwen, Nathaniel E. Putzig, Donna Viola, Sarah Sutton, Erin Clark, John W. Holt
- ↑ Steep Slopes on Mars Reveal Structure of Buried Ice. NASA Press Release. 11 January 2018.
- ↑ Ice cliffs spotted on Mars. Science News. Paul Voosen. 11 January 2018.
- ↑ Dundas, E., et al. 2018. Exposed subsurface ice sheets in the martian mid-latitudes. Science. 359. 199.
- ↑ http://spaceref.com/mars/steep-slopes-on-mars-reveal-structure-of-buried-ice.html
- ↑ https://www.uahirise.org/ESP_047247_1150
- ↑ http://www.uahirise.org/ESP_046359_1250
- ↑ Soare, E., et al. 2019.
- ↑ Possible (closed system) pingo and ice-wedge/thermokarst complexes at the mid latitudes of Utopia Planitia, Mars. Icarus. https://doi.org/10.1016/j.icarus.2019.03.010
- ↑ https://marsed.asu.edu/mep/ice/chaos-terrain
- ↑ https://link.springer.com/referenceworkentry/10.1007%2F978-1-4614-9213-9_46-2
- ↑ https://www.uahirise.org/hipod/PSP_008311_1835
- ↑ https://www.uahirise.org/hipod/PSP_008244_2645
- ↑ Schorghofer, Norbert (2008). "Temperature response of Mars to Milankovitch cycles". Geophysical Research Letters. 35 (18): doi:10.1029/2008GL034954.
- ↑ ouma | first1 = J. | last2 = Wisdom | first2 = J. | year = 1993 | title = The Chaotic Obliquity of Mars | url = | journal = Science | volume = 259 | issue = 5099| pages = 1294–1297 |
- ↑ Laskar | first1 = J. | last2 = Correia | first2 = A. | last3 = Gastineau | first3 = M. | last4 = Joutel | first4 = F. | last5 = Levrard | first5 = B. | last6 = Robutel | first6 = P. | year = 2004 | title = Long term evolution and chaotic diffusion of the insolation quantities of Mars | url = | journal = Icarus | volume = 170 | issue = 2| pages = 343–364 |
- ↑ Kreslavsky, M., J. Head, J. 2002. Mars: Nature and evolution of young, latitude-dependent water-ice-rich mantle. Geophys. Res. Lett. 29, doi:10.1029/ 2002GL015392.
- ↑ https://www.uahirise.org/PSP_008391_1790
- ↑ Rates and mechanisms of chemical weathering of ferromagnesian silicate minerals on Mars | date = 1993 | last1 = Burns | first1 = Roger G | journal = Geochimica et Cosmochimica Acta | volume = 57 | issue = 19 | pages = 4555–4574 |
- ↑ {{cite journal | doi = 10.1029/92JE02055 | title = Rates of Oxidative Weathering on the Surface of Mars | date = 1993 | last1 = Burns | first1 = Roger G. | last2 = Fisher | first2 = Duncan S. | journal = Journal of Geophysical Research | volume = 98 | issue = E2 | pages = 3365–3372 |
- ↑ Hurowitz | first1 = J. A. | last2 = Fischer | first2 = W. W. | last3 = Tosca | first3 = N. J. | last4 = Milliken | first4 = R. E. | year = 2010 | title = Origin of acidic surface waters and the evolution of atmospheric chemistry on early Mars | url = https://authors.library.caltech.edu/18444/2/ngeo831-s1.pdf%7C journal = Nat. Geosci. | volume = 3 | issue = 5| pages = 323–326 | doi = 10.1038/ngeo831 |
- ↑ https://www.uahirise.org/PSP_009501_1755
- ↑ https://www.jpl.nasa.gov/edu/learn/video/mars-in-a-minute-how-did-mars-get-such-enormous-mountains/
- ↑ https://astronomy.com/magazine/ask-astro/2013/08/valles-marineris
- ↑ A. Broquet, J. C. Andrews-Hanna. Geophysical evidence for an active mantle plume underneath Elysium Planitia on Mars. Nature Astronomy, 2022; DOI: 10.1038/s41550-022-01836-3
- ↑ https://www.sciencedaily.com/releases/2022/12/221205121545.htm
- ↑ https://www.nature.com/articles/s41550-022-01836-3
- ↑ Sullivan, R. et al. 2001. Mass Movement Slope Streaks Imaged by the Mars Orbiter Camera. J. Geophys. Res., 106(E10), 23,607–23,633.
- ↑ https://en.wikipedia.org/wiki/Micrometre
- ↑ A. Bhardwaj, L. Sam, F.J. Martín-Torres, M.P. Zorzano. 2019. Are slope streaks indicative of global-scale aqueous processes on contemporary Mars? Rev. Geophys. 10.1029/2018RG000617
- ↑ https://www-sciencedirect-com.wikipedialibrary.idm.oclc.org/science/article/pii/S0019103520303249#bb0020
- ↑ Heyer, T., et al. 2020. Dust devil triggering of slope streaks on Mars. Icarus. Icarus Volume 351. 113951
- ↑ Kaylan J. Burleigh, Henry J. Melosh, Livio L. Tornabene, Boris Ivanov, Alfred S. McEwen, Ingrid J. Daubar. Impact air blast triggers dust avalanches on Mars" Icarus 2012; 217 (1) 194
- ↑ http://redplanet.asu.edu/
- ↑ http://phys.org/news/2011-12-meteorite-shockwaves-trigger-avalanches-mars.html
- ↑ https://www.uahirise.org/ESP_042201_1715
- ↑ http://hirise.lpl.arizona.edu/PSP_005383_1255
- ↑ https://www.compadre.org/osp/items/detail.cfm?ID=9757
- ↑ https://www.compadre.org/osp/items/detail.cfm?ID=7305
- ↑ NASA/Goddard Space Flight Center. "New gravity map gives best view yet inside Mars." ScienceDaily. ScienceDaily, 21 March 2016. https://www.sciencedaily.com/releases/2016/03/160321154013.htm.
- ↑ Antonio Genova, Sander Goossens, Frank G. Lemoine, Erwan Mazarico, Gregory A. Neumann, David E. Smith, Maria T. Zuber. Seasonal and static gravity field of Mars from MGS, Mars Odyssey and MRO radio science. Icarus, 2016; 272: 228 DOI: 10.1016/j.icarus.2016.02.05
- ↑ https://mars.jpl.nasa.gov/mgs/msss/camera/images/dune_defrost_6_2001/
- ↑ SPRING DEFROSTING OF MARTIAN POLAR REGIONS: MARS GLOBAL SURVEYOR MOC AND TES MONITORING OF THE RICHARDSON CRATER DUNE FIELD, 1999–2000. K. S. Edgett, K. D. Supulver, and M. C. Malin, Malin Space Science Systems, P.O. Box 910148, San Diego, CA 92191-0148, USA.
- ↑ K.-Michael Aye, K., et al. PROBING THE MARTIAN SOUTH POLAR WINDS BY MAPPING CO2 JET DEPOSITS. 49th Lunar and Planetary Science Conference 2018 (LPI Contrib. No. 2083). 2841.pdf
- ↑ http://www.jpl.nasa.gov/news/news.php?release=2013-034
- ↑ https://www.uahirise.org/
- ↑ http://spaceref.com/mars/how-gas-carves-channels-on-mars.html
- ↑ http://themis.asu.edu/news/gas-jets-spawn-dark-spiders-and-spots-mars-icecap
- ↑ http://mars.jpl.nasa.gov/multimedia/images/2016/possible-development-stages-of-martian-spiders
- ↑ http://spaceref.com/mars/growth-of-a-martian-trough-network.html
- ↑ Benson, M. 2012. Planetfall: New Solar System Visions
- ↑ http://www.astrobio.net/topic/solar-system/mars/spiders-invade-mars/
- ↑ Kieffer H, Christensen P, Titus T. 2006 Aug 17. CO2 jets formed by sublimation beneath translucent slab ice in Mars' seasonal south polar ice cap. Nature: 442(7104):793-6.
- ↑ Portyankina, G., et al. 2017. Present-day erosion of Martian polar terrain by the seasonal CO2 jets. Icarus: 282, 93-103.
- ↑ Portyankina, G., et al. 2019. How Martian araneiforms get their shapes: morphological analysis and diffusion-limited aggregation model for polar surface erosion Icarus. https://doi.org/10.1016/j.icarus.2019.02.032
- ↑ Thomas,P., M. Malin, P. James, B. Cantor, R. Williams, P. Gierasch South polar residual cap of Mars: features, stratigraphy, and changes Icarus, 174 (2 SPEC. ISS.). 2005. pp. 535–559. http://doi.org/10.1016/j.icarus.2004.07.028
- ↑ Thomas, P., P. James, W. Calvin, R. Haberle, M. Malin. 2009. Residual south polar cap of Mars: stratigraphy, history, and implications of recent changes Icarus: 203, 352–375 http://doi.org/10.1016/j.icarus.2009.05.014
- ↑ Thomas, P., W.Calvin, P. Gierasch, R. Haberle, P. James, S. Sholes. 2013. Time scales of erosion and deposition recorded in the residual south polar cap of mars Icarus: 225: 923–932 http://doi.org/10.1016/j.icarus.2012.08.038
- ↑ Thomas, P., W. Calvin, B. Cantor, R. Haberle, P. James, S. Lee. 2016. Mass balance of Mars’ residual south polar cap from CTX images and other data Icarus: 268, 118–130 http://doi.org/10.1016/j.icarus.2015.12.038
- ↑ Buhler, Peter, Andrew Ingersoll, Bethany Ehlmann, Caleb Fassett, James Head. 2017. How the martian residual south polar cap develops quasi-circular and heart-shaped pits, troughs, and moats. Icarus: 286, 69-9.
- ↑ Vincendon, M. 2015. JGR:120, 1859–1879.
- ↑ Pilorget | first1 = C. | last2 = Forget | first2 = F. | year = 2016 | title = Formation of gullies on Mars by debris flows triggered by CO2 sublimation | url = | journal = Nature Geoscience | volume = 9 | issue = | pages = 65–69 | doi = 10.1038/ngeo2619 |
- ↑ Schorghofer, N., K. Edgett. 2005. Seasonal surface frost at low latitudes on Mars. Icarus: 180, 321-334.
- ↑ Harrington |first=J.D. |last2=Webster |first2=Guy |title=RELEASE 14-191 – NASA Spacecraft Observes Further Evidence of Dry Ice Gullies on Mars |url=http://www.nasa.gov/press/2014/july/nasa-spacecraft-observes-further-evidence-of-dry-ice-gullies-on-mars |
- ↑ CNRS. "Gullies on Mars sculpted by dry ice rather than liquid water." ScienceDaily. ScienceDaily, 22 December 2015. www.sciencedaily.com/releases/2015/12/151222082255.htm
- ↑ http://www.skyandtelescope.com/astronomy-news/martian-gullies-triggered-by-exploding-dry-ice-122320158
- ↑ https://www.uahirise.org/ESP_057071_1890
- ↑ https://www.uahirise.org/ESP_057071_1890
- ↑ https://www.uahirise.org/ESP_057071_1890
- ↑ http://www.lpi.usra.edu/meetings/lpsc2012/pdf/1598.pdf%7Cformat=PDF%7Ctype=conference paper|title=Investigations of transverse aeolian ridges on Mars|first1=Daniel C.|last1=Berman|first2=Matthew R.|last2=Balme|year=2012|publisher=Lunar and Planetary Science Conference
- ↑ http://www.uahirise.org/ESP_042625_1655
- ↑ Berman, D., et al. 2018. High-resolution investigations of Transverse Aeolian Ridges on Mars: Icarus: 312, 247-266.
- ↑ Pascuzzo, A., et al. 2019. The formation of irregular polygonal ridge networks, Nili Fossae, Mars: Implications for extensive subsurface channelized fluid flow in the Noachian. Icarus: 319, 852-868
- ↑ Kerber, L., et al. 2017. Polygonal ridge networks on Mars: Diversity of morphologies and the special case of the Eastern Medusae Fossae Formation. Icarus. Volume 281. Pages 200-219
- ↑ https://www.uahirise.org/hipod/PSP_008189_2080
- ↑ https://www.uahirise.org/hipod/ESP_077982_1920
- ↑ https://news.asu.edu/20220405-citizen-scientists-help-map-ridge-networks-may-hold-records-ancient-groundwater-mars
- ↑ Khuller, A., et al. 2022. Irregular polygonal ridge networks in ancient Noachian terrain on Mars. Icarus. 374. 114833
External links
- https://mail.google.com/mail/u/0/?tab=wm&pli=1#inbox/FMfcgzGrbHtSWzJjdRzftxgXCrbxXNnK Water on Mars - A Literature Review" by Mohammad Nazari-Sharabian
- Features of Mars with HiRISE under HiWish program Shows nearly all major features discovered on Mars. This would be good for teachers covering Mars.
- Martian Ice - Jim Secosky - 16th Annual International Mars Society Convention
- Martian Geology - Jim Secosky - 16th Annual International Mars Society Convention
- Walks on Mars - Jim Secosky - 16th Annual International Mars Society Convention
- Jawin, E.,et al. 2018. Transient post-glacial processes on Mars: Geomorphologic evidence for a paraglacial period. Icarus. Volume 309. Pages 187-206
See Also
- Geography of Mars
- Glaciers on Mars
- High Resolution Imaging Science Experiment (HiRISE)
- Layers on Mars
- Martian features that are signs of water ice
- Martian gullies
- Rivers on Mars
- Sublimation
- Sublimation landscapes on Mars
Recommended reading
- Head, J., et al. 2023. GEOLOGICAL AND CLIMATE HISTORY OF MARS: IDENTIFICATION OF POTENTIAL WARM AND
WET CLIMATE ‘FALSE POSITIVES’. 54th Lunar and Planetary Science Conference 2023 (LPI Contrib. No. 2806). 1731.pdf
- Grotzinger, J., R. Milliken (eds.). 2012. Sedimentary Geology of Mars. Tulsa: Society for Sedimentary Geology.
- Kieffer, H., et al. (eds) 1992. Mars. The University of Arizona Press. Tucson
- history.nasa.gov/SP-4212/ch11
- Lorenz, R. 2014. The Dune Whisperers. The Planetary Report: 34, 1, 8-14
- Lorenz, R., J. Zimbelman. 2014. Dune Worlds: How Windblown Sand Shapes Planetary Landscapes. Springer Praxis Books / Geophysical Sciences.