Difference between revisions of "What Mars Actually Looks Like!"
Suitupshowup (talk | contribs) (added image) |
Suitupshowup (talk | contribs) m |
||
Line 131: | Line 131: | ||
[[File:ESP 045085 2205flowlabeled.jpg|thumb|300px|center|Labeled view of Lineated Valley Flow and glacier]] | [[File:ESP 045085 2205flowlabeled.jpg|thumb|300px|center|Labeled view of Lineated Valley Flow and glacier]] | ||
− | |||
Line 304: | Line 303: | ||
==Dust Devil Tracks== | ==Dust Devil Tracks== | ||
− | |||
− | |||
Dust devil tracks can be very beautiful. They are made by giant [[dust devils]] removing bright colored dust from the Martian surface; consequently exposing a dark layer.<ref>https://www.uahirise.org/ESP_058427_1080</ref> Dust devils on Mars have been photographed both from the ground and from orbit. They have even blown dust off the solar panels of two Rovers on Mars, thereby greatly extending their useful lifetime.<ref>http://marsrovers.jpl.nasa.gov/gallery/press/spirit/20070412a.html Mars Exploration Rover Mission: Press Release Images: Spirit. Marsrovers.jpl.nasa.gov</ref> The dust devils can be 650 meters high and 50 meters across.<ref> https://www.uahirise.org/ESP_061787_2140</ref> The pattern of the tracks has been shown to change every few months.<ref>http://hirise.lpl.arizona.edu/PSP_005383_1255</ref> | Dust devil tracks can be very beautiful. They are made by giant [[dust devils]] removing bright colored dust from the Martian surface; consequently exposing a dark layer.<ref>https://www.uahirise.org/ESP_058427_1080</ref> Dust devils on Mars have been photographed both from the ground and from orbit. They have even blown dust off the solar panels of two Rovers on Mars, thereby greatly extending their useful lifetime.<ref>http://marsrovers.jpl.nasa.gov/gallery/press/spirit/20070412a.html Mars Exploration Rover Mission: Press Release Images: Spirit. Marsrovers.jpl.nasa.gov</ref> The dust devils can be 650 meters high and 50 meters across.<ref> https://www.uahirise.org/ESP_061787_2140</ref> The pattern of the tracks has been shown to change every few months.<ref>http://hirise.lpl.arizona.edu/PSP_005383_1255</ref> | ||
Line 315: | Line 312: | ||
[[File:PIA26074-MarsPerseveranceRover-Whirlwind-20230830.gif|thumb|center|300px|Dust devil as seen by Perseverance rover It is 5 times taller than the Empire State Building | [[File:PIA26074-MarsPerseveranceRover-Whirlwind-20230830.gif|thumb|center|300px|Dust devil as seen by Perseverance rover It is 5 times taller than the Empire State Building | ||
− | [[ | + | [[File:dust_devils.gif|thumb|right|300px|Dust devils photographed by Mars Rover Spirit]] |
<gallery class="center" widths="380px" heights="360px"> | <gallery class="center" widths="380px" heights="360px"> |
Revision as of 13:55, 14 October 2023
Article written by Jim Secosky. Jim is a retired science teacher who has used the Hubble Space Telescope, the Mars Global Surveyor, and HiRISE.
Almost all of the sites that we have landed on Mars with spacecraft have been to the most drab and boring places on the planet. This was done to ensure a safe landing. This article will display many of the more exciting landscapes using HiRISE images. HiRISE images can show detail down to the size of a small kitchen table. With HiRISE we frequently even see spacecraft that have landed on the surface. Many of the scenes shown here are about one would see at the height of a helicopter.
Most of the HiRISE images here were obtained through the HiWish program, a program where anyone could suggest places to be imaged with HiRISE. To obtain the images, I studied wide angle CTX images to find sites that could contain interesting features. I was lucky that many of my suggestions were photographed, and I was able to gather them together for this article.
Contents
- 1 Viking 1
- 2 Viking 2
- 3 Mars Pathfinder
- 4 Spirit Rover
- 5 Opportunity Rover
- 6 Phoenix
- 7 Curiosity Rover
- 8 Dunes
- 9 Layers
- 10 Glaciers
- 11 Gullies
- 12 Channels
- 13 Inverted relief
- 14 Troughs
- 15 Craters
- 16 Scalloped Terrain
- 17 Brain Terrain
- 18 Ribbed terrain
- 19 Linear Ridge Networks
- 20 Yardangs
- 21 Dust Devil Tracks
- 22 Dark Slope Streaks
- 23 Lava
- 24 Mud Volcanoes
- 25 Rootless cones
- 26 Honeycomb Terrain
- 27 Fractured Surface and Blocks
- 28 Fractured Ground
- 29 Dipping layers
- 30 Boulders
- 31 Hollows
- 32 Mesas
- 33 Landslides
- 34 Latitude Dependent Mantle
- 35 Exhumed craters
- 36 Swiss Cheese Terrain
- 37 Ice Cap Layers
- 38 Spiders
- 39 Polygonal Patterned Ground
- 40 Notes about pictures
- 41 References
- 42 See Also
- 43 Further reading
- 44 External links
Viking 1
Viking 1 was the first successful spacecraft to land on Mars. It landed on July 20, 1976 at 22.27 N and 47.95 W (312.05 E). July 20th was also the date when we first landed on the moon in 1969.
Viking 2
Viking 2 landed on September 3, 1976 at 47.64 N and 275.71 W (84.29 E).
Mars Pathfinder
The Mars Pathfinder landed on July 4, 1997 at 19 degrees 7’ 48” in Ares Vallis.
Spirit Rover
The Spirit Rover landed on January 4, 2004 at 14.5684 S and 175.472636 E (184.527364 W).
Opportunity Rover
The Opportunity Rover landed on January 25, 2004 at 1.9462 S and 354.4734 E (5.5268 W).
Wide view of Perserverance Valley taken with Opportunity Rover High points visible on the rim of Endeavour Crater include "Winnemucca" on the left and "Cape Tribulation" on the right. Winnemucca is part of the "Cape Byron" portion of the crater rim. The horizon at far right extends across the floor of Endeavour Crater, which is about 14 miles (22 kilometers) in diameter.
Phoenix
Phoenix landed in the far North of Mars on May 25, 2008 at 68.22 N and 125.7 W (234.3 E) in Vastitas Borealis.
Curiosity Rover
The Curiosity Rover landed on August 6, 2012 at Gale Crater in Aeolis Palus at 4.5895 S and 137.4417 E (222.5583 W). By this time scientists were able to be more precise with their landings, so Curiosity has been able to get views of Mars that are pretty exciting.
What follows are a few pictures of the many different scenes that we have studied with powerful cameras on board the Mars Reconnaissance Orbiter that has been going around Mars for over 10 years.
Dunes
The Martian surface displays many beautiful dark dunes. For many years, scientists thought dark dunes were composed of the grains of sand from the volcanic rock basalt; this was confirmed by rovers on the surface.[1] The dunes are covered by a seasonal carbon dioxide frost that forms in early autumn and remains until late spring.
The presence of dunes on Mars and the observations that they do change is clear proof that there is air on Mars. However, we must remember that its atmosphere is only about 1 % as dense as the Earth's. Hence, a wind speed of a 60-mph storm on Mars would feel more like 6 mph (9.6 km/hr).[2]
Colorful dunes in the Mare Tyrrhenum quadrangle[5]
Dunes This image was named picture of the day for July 25, 2021
Layers
Many places on Mars show rocks arranged in layers. Volcanoes, wind, or water can produce layers.[6] Layers can be hardened by the action of groundwater.
Layers and fault in Firsoff Crater in Oxia Palus quadrangle, as seen by HiRISE under HiWish program
Glaciers
There are large areas on Mars that contain what is thought to be ice moving under a cover of debris. A few meters of debris can preserve ice for long periods of time.[7]
Several types of landforms have been identified as probably dirt and rock debris covering huge deposits of ice.[8] [9] [10] [11] Concentric crater fill (CCF) contains dozens to hundreds of concentric ridges that are caused by the movements of sometimes hundreds of meter thick accumulations of ice in craters.[12] [13] Lineated valley fill (LVF) are lines of ridges in valleys.[14] [15] [16] These lines may have developed as other glaciers moved down valleys. Some of these glaciers seem to come from material sitting around mesas and buttes.[17] Lobate debris aprons (LDA) is the name given to these glaciers. All of these features that are believed to contain large amounts of ice are found in the mid-latitudes in both the Northern and Southern hemispheres.[18] [19] [20]
Gullies
Martian gullies are networks of narrow channels and their associated downslope deposits, found on steep slopes. A high concentration occurs near 40 degrees north and south of the equator. Usually, each gully has an alcove at its head, a fan-shaped apron at its base, and a channel linking the two.[21] They are believed to be relatively young because they have few, if any craters. They were believed to be caused by recent running water, but with more observations other ideas emerged. In summary of our present understanding of gullies it can be said: A number of studies have demonstrated that gullies are being modified on present day Mars. [22] [23] [24] [25] Today, liquid water cannot exist on the Red planet because the both the pressure and the temperature are too low. Researchers have proposed other mechanisms that could account for gully formation without liquid water.[26] Most involve dry ice (solid carbon dioxide) accumulating during cold seasons and then changing to a gas in the spring. The gas coming off could start material moving down slopes. The gas mixed with sand and other debris could act like water to erode channels. Also, pieces of dry ice could easily side down due to the lubricating effect of gas coming off the dry ice. However, one wonders if these processes could account for the formation of all the gullies. Maybe, liquid water was sometimes necessary, especially to move large boulders. A study of over 700 sites, published in 2022 in Icarus, concluded that liquid water would not have been needed. During the duration of the study many large boulders were moved—one being 5 meters across. Many types of changes were seen in gullies. Some channels were extended, new channels were formed, and other channels were filled with new debris.[27] [28] Perhaps, some water was involved in the past, but all the gullies seen today could have been made without water.
Gullies in Phaethontis quadrangle Ridges at the end of the gullies may be the remains of old glaciers.[29]
Channels
There are thousands of channels that were probably caused by running water in the past on Mars. Some are large; some are tiny.[30] [31] [32] [33] [34]
Streamlined forms in wide channel These were shaped by running water.[35]
Inverted relief
Some places on Mars show inverted relief. In these locations, a stream bed may be a raised feature, instead of a valley. Inverted former stream channels may be caused by the deposition of large rocks, cementation, or maybe by lava moving down the channel. In either case later erosion would erode the surrounding land and leave the old channel as a raised ridge because the ridge would be more resistant to erosion. The image below, taken with HiRISE show curved ridges that are old channels that have become inverted. They have the shape of streams but are above ground.[36]
Troughs
The great weight of several huge volcanoes on Mars has stretched the crust and made it break into cracks called, “troughs” or “fossae.” Some of them show evidence that lava and/or water have come out of them in the past. They can be very long.[37] [38] [39]
Craters
Most of the surface of Mars is over a billion years old. Because Mars has not had active plate tectonics for a very long time (if it ever had active plate tectonics), impact craters stay for a long time. So, impact craters are a major surface feature. There is a rich variety of craters on the planet.[40] [41] We have found that Mars is hit by 200 impacts/year.[42] [43] [44]
Young crater with bright ejecta in the Phoenicis Lacus quadrangle as seen by HiRISE under HiWish program The impact reached down to a layer that is light-toned. That light-toned material was then deposited on a dark surface.
Group of secondary craters These are formed from material that is blasted way up in the air from the impact.[45]
Layered mound in crater Layers represent material that once covered a wide area. Mound was shaped by winds.[46]
New, small crater We have detected many new craters on Mars that have impacted the planet since good cameras have orbited the planet. To see before and after photos of a new impact go to https://static.uahirise.org/images/2020/details/cut/ESP_062948_2175.gif
Scientists love to study central peaks of craters because they contain samples of material from deep under a surface. Durning an impact, the ground is pushed down. It then rebounds and brings up rocks from deep underground.[49]
Scalloped Terrain
Scalloped topography is common in the mid-latitudes of Mars, between 45° and 60° north and south. It is especially prominent in the region of Utopia Planitia.[50] [51] Such topography consists of shallow, rimless depressions with scalloped edges, commonly referred to as "scalloped depressions" or simply "scallops". Scalloped depressions can be isolated or clustered and sometimes seem to coalesce. The usual scalloped depression displays a gentle equator-facing slope and a steeper pole-facing scarp.[52] [53] [54] Scalloped topography may be of great importance for future colonization of Mars because it may point to deposits of pure ice.[55] [56]
Brain Terrain
Brain terrain is a region of maze-like ridges 3–5 meters high. A person could wander between these ridges like a rat in a maze. Some ridges may consist of an ice core, so they may be sources of water for future colonists.[57]
Labeled picture of open and closed brain terrain in the Ismenius Lacus quadrangle Closed-cell brain terrain may still contain an ice core.[58]
Ribbed terrain
Ribbed terrain forms as ice leaves the ground along cracks in a process called " sublimation." Much of the ground is ice so that when the ice disappears the ground collapses.[59]
Linear Ridge Networks
Close view of ridge network, as seen by HiRISE under HiWish program
This terrain appears over much of the planet. However, there is a heavy concentration of these features, also called irregular polygonal ridge networks, in the Nili Fossae region.[60] These networks consist of groups of narrow ridges that often meet at close to right angles. We are not sure of how it originated.[61] They may have been caused by fluids moving into cracks that were created by impacts. The fluids then became hard and erosion resistant.[62] [63] [64] [65] [66] [67] [68] We are not totally sure of the exact ways these ridges were created. Over 14,000 people from around the world helped map them, so that scientists could better understand them. Some ridges contain clays, so water may have been involved in their formation because clays need water to form.[69] [70]
Yardangs
Yardangs form from fine-grained material. They are shaped by the wind and show the direction of the prevailing winds. Much of this fine-grained material probably has its origin in the many large volcanoes on the planet. Yardangs are especially common in what's called the "Medusae Fossae Formation." This formation is found in the Amazonis quadrangle and near the equator.[71] Because they exhibit very few impact craters they are believed to be relatively young.[72]
Dust Devil Tracks
Dust devil tracks can be very beautiful. They are made by giant dust devils removing bright colored dust from the Martian surface; consequently exposing a dark layer.[73] Dust devils on Mars have been photographed both from the ground and from orbit. They have even blown dust off the solar panels of two Rovers on Mars, thereby greatly extending their useful lifetime.[74] The dust devils can be 650 meters high and 50 meters across.[75] The pattern of the tracks has been shown to change every few months.[76]
Dust devils are common.[77] One team of researchers have calculated that on average 1 dust devil happens every sol (day on Mars) for each square kilometer.[78] [79] In the first 216 Martian days (Sols), the Perseverance Rover in Jezero Crater found that at least four dust devils passed Perseverance on a typical Martian day and that more than one per hour passes by during a peak hourlong period just after noon.[80] [81] [82] Perseverance Rover recorded a tall dust devil in the distance on Aug. 30, 2023. Scientists calculated that the dust devil was about 2.5 miles (4 kilometers) away and was moving east to west at about 12 mph (19 kph). Its width was about 200 feet (60 meters). Even though only the bottom 387 feet (118 meters) of the devil was visible in the camera frame, scientists estimated its total height at about 1.2 miles (2 kilometers) using the dust devil's shadow.[83]

Dark Slope Streaks
Dark slope streaks are avalanche-like features common on dust-covered slopes, especially in the equatorial regions.[84] These streaks have never been observed on the Earth.[85] They form in relatively steep terrain, such as along cliffs and crater walls.[86] The darkest streaks are only about 10% darker than their surroundings. The streaks seem much darker because of contrast enhancement in the image processing.[87] Dry ice accumulates just under the surface during cold Martian nights and then changes to a gas in the morning. That gas creates enough wind to disturb dust particles and send them down steep slopes. As the bright dust slides down it reveals the underlying dark volcanic rocks. This process was discovered by measuring temperatures in the area. At the recorded temperatures, carbon dioxide from the air should have frozen on the surface, but it was not visible. It was concluded that the dry ice was forming just under the surface rather than on top..[88] [89]Lava
Large areas of Mars are covered with lava flows.[90] [91] [92] [93] Lava flows can also move around an create what appear to be layers, especially if it fluid like water. Basalt flows can often be that way.[94]Mud Volcanoes
Mud volcanoes are very common in the Mare Acidalium quadrangle. Because they bring up mud from underground, they may hold evidence of life.[95] Being underground the mud was protected from radiation on the surface. Methane has been detected on Mars; methane may be produced by certain bacteria. Some scientists speculate that methane may come from mud volcanoes.[96]Rootless cones
Rootless Cones are believed to be caused by lava flowing over ice or ground containing ice.[97] [98] Heat from the lava causes the ice to quickly change to steam which blows out a ring or cone. Some of the forms do not have the shape of rings or cones because maybe the lava moved too quickly; thereby not allowing a complete cone shape to form.Honeycomb Terrain
Honeycomb terrain is found on parts of the floor of Hellas Planitia. It may be due to rising bodies of ice followed by erosion.[99] [100] [101]Fractured Surface and Blocks
In many places on Mars bedrock breaks up into large blocks. Sometimes the blocks form what look like perfect cubes. Although one may think these shapes had to be made by intelligent aliens, this is a natural process. The salt you put on your food also breaks up into cubes. Check your salt out with a magnifying glass.Fractured Ground
Some places on Mars break up with large fractures that create a terrain with mesas and valleys. Some of these can be quite pretty.Dipping layers
Groups of layers that are tilted are common in some areas of Mars. They represent material that once covered a wide area.[102] [103] The layers may be related to changes in the climate in the past. They may have been shaped by the wind.Boulders
Much of the surface of Mars is covered with hard, basalt volcanic rock. When the rock breaks down it often forms large boulders the size of houses.Hollows
Some places on Mars have surfaces that are covered with hollows. Sometimes they form large holes, sometimes curved canyons. They can be pretty and would be fun to explore on foot in the future. This terrain may have developed from what has been called ribbed terrain. Either way, these scenes were caused as ice left the ground.Mesas
Many, large areas of Mars have eroded such that there are many mesas. Some show layers. Mesas show how the kind of material that covered a wide area. Mesas are what are left after the ground is mostly eroded.Landslides
Mars shows various mass movements like landslides. There are many steep slopes for material to move down, especially in craters and canyons.Latitude Dependent Mantle
Latitude Dependent Mantle is very common in certain latitudes.[104] It often appears as a smooth covering. A certain percentage of it consists of ice. It may be a major source of water for future colonists because it has a widespread distribution. Sometimes mantle displays layers because it was deposited at different times.Exhumed craters
Exhumed craters seem to be in the process of being uncovered.[105] [106] The surface of Mars is very old. Places have been covered, uncovered, and covered again by sediments. The pictures below show a crater that is being exposed by erosion. When a crater forms, it will destroy what's under it. In the example below, only part of the crater is visible. Had the crater been created after the layered feature, it would have removed part of the feature and we would see the entire crater.Swiss Cheese Terrain
Parts of Mare Australe show pits that make the surface look like Swiss cheese.[107] [108] [109] [110] These pits are in a 1-10 meter thick layer of dry ice that lies on a much larger water ice cap. These circular pits have steep walls that work to focus sunlight, thereby increasing erosion. For a pit to develop, a steep wall of about 10 cm and a length of over 5 meters in necessary.[111]Ice Cap Layers
The northern ice cap of Mars displays many layers of ice that accumulated when the climate changed. These are visible when there is a canyon in the ice. The climate of Mars changes greatly due to the large changes in the tilt of Mars. Mars does not have a large moon to stabilize its' tilt.Spiders
The official name for spiders is "araneiforms."As the temperature goes up in the spring, pressurized carbon dioxide gas and dark dust are released from under slabs of ice.[112] This results in the appearance of dark plumes that are often blown in one direction by local winds. This dust darkens channels under the ice and forms dark shapes that resemble spiders.[113] [114] [115] [116] This process was demonstrated in laboratory simulations involving slabs of dry ice placed on glass spheres of different sizes.[117] [118] [119]Polygonal Patterned Ground
Many surfaces on Mars display “polygonal patterned ground.” The polygons can be of different shapes and sizes. They are believed to be caused by ice in the ground.[120] Like permafrost regions on Earth, this permanently frozen water is still active. With the changing seasons, alternate cooling and warming causes the ice-cemented soil to contract and expand. With the right conditions, cracks are made into the hard frozen ground releasing the stresses caused by contraction. Over long periods of cyclic cracking, a honeycomb-like polygonal pattern arises.[121] The patterns formed may yet be another marker for underground ice that could be used by future colonists. Before we land crews on Mars, we may very well have detailed maps for where the colonists can obtain water.Notes about pictures
Most pictures from spacecraft have some sort of enhancement. For many views of Mars there is not much contrast, so the contrast is enhanced in a process known as stretching. In that process the darkest parts are set to black while the lightest parts are set to be white. The colors for HiRISE images are different than the human eye would see. HiRISE only sees in only 3 colors and sometimes infrared is used rather than red.[122] [123] Displaying colors in this way allows us to better identify rocks and minerals. HiRISE images are about 5 km wide with a 1 km wide band in the center that is in color.[124]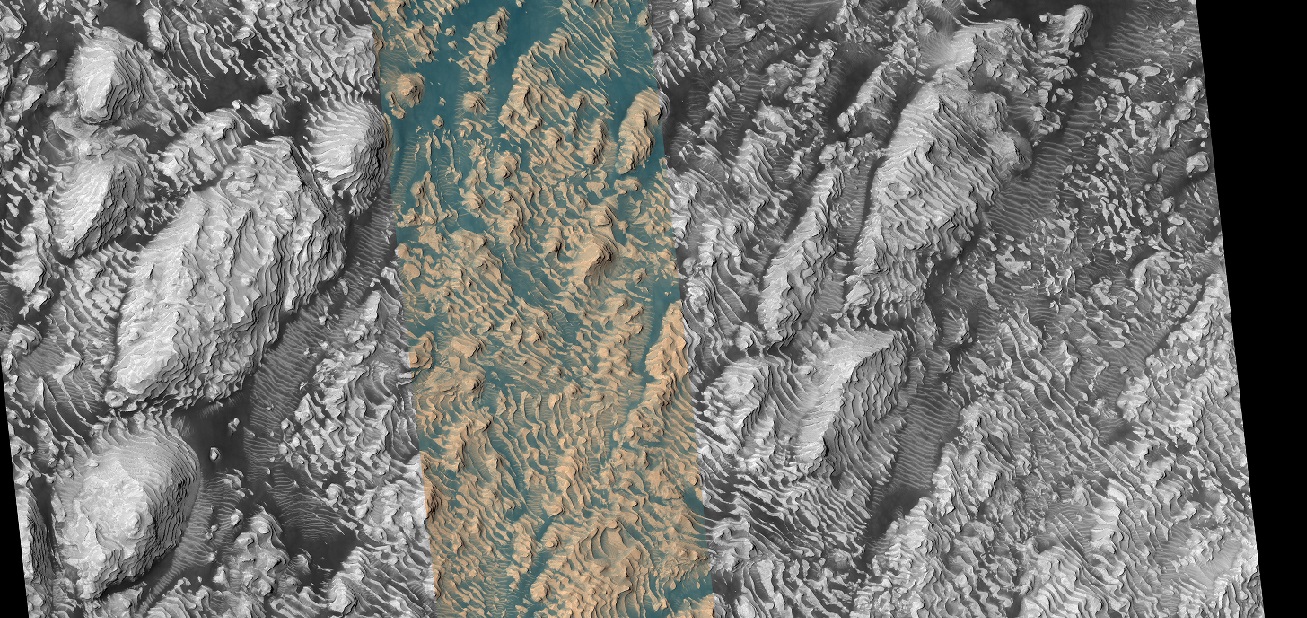
References
- ↑ Lorenz, R. and J. Zimbelman. 2014. Dune Worlds How Windblown Sand Shapes Planetary Landscapes. Springer. NY.
- ↑ https://www.space.com/30663-the-martian-dust-storms-a-breeze.html
- ↑ https://www.uahirise.org/ESP_057071_1890
- ↑ https://www.uahirise.org/ESP_057071_1890
- ↑ https://www.uahirise.org/ESP_057071_1890
- ↑ url=http://hirise.lpl.arizona.edu?PSP_008437_1750 |title=HiRISE | High Resolution Imaging Science Experiment |publisher=Hirise.lpl.arizona.edu?psp_008437_1750
- ↑ Head, J. W.; et al. (2006). "Extensive valley glacier deposits in the northern mid-latitudes of Mars: Evidence for Late Amazonian obliquity-driven climate change". Earth and Planetary Science Letters. 241 (3): 663–671.
- ↑ Head, J. and D. Marchant. 2006. Evidence for global-scale northern mid-latitude glaciation in the Amazonian period of Mars: Debris-covered glacial and valley glacial deposits in the 30 - 50 N latitude band. Lunar. Planet. Sci. 37. Abstract 1127
- ↑ Head, J. and D. Marchant. 2006. Modifications of the walls of a Noachian crater in Northern Arabia Terra (24 E, 39 N) during northern mid-latitude Amazonian glacial epochs on Mars: Nature and evolution of Lobate Debris Aprons and their relationships to lineated valley fill and glacial systems. Lunar. Planet. Sci. 37. Abstract 1128
- ↑ Head, J., et al. 2006. Extensive valley glacier deposits in the northern mid-latitudes of Mars: Evidence for the late Amazonian obliquity-driven climate change. Earth Planet. Sci. Lett. 241. 663-671
- ↑ Head, J., et al. 2006. Modification if the dichotomy boundary on Mars by Amazonian mid-latitude regional glaciation. Geophys. Res Lett. 33
- ↑ Garvin, J. et al. 2002. Lunar Planet. Sci: 33. Abstract # 1255.
- ↑ http://photojournal.jpl.nasa.gov/catalog/PIA09662
- ↑ Carr, M. 2006. The Surface of Mars. Cambridge University Press. ISBN|978-0-521-87201-0
- ↑ Squyres, S. 1978. Martian fretted terrain: Flow of erosional debris. Icarus: 34. 600-613.
- ↑ Levy, J. et al. 2007. Lineated valley fill and lobate debris apron stratigraphy in Nilosyrtis Mensae, Mars: Evidence for phases of glacial modification of the dichotomy boundary. J. Geophys. Res. 112
- ↑ Baker, D., et al. 2009. Flow patterns of lobate debris aprons and lineated valley fill north of Ismeniae Fossae, Mars: Evidence for extensive mid-latitude glaciation in the Late Amazonian. Icarus: 207. 186-209.
- ↑ Marchant, D. and J. Head. 2007. Antarctic dry valleys: Microclimate zonation, variable geomorphic processes, and implications for assessing climatic change on Mars. Icarus: 192.187-222
- ↑ Dickson, J., et al. 2008. Late Amazonian glaciation at the dichotomy boundary on Mars: Evidence for glacial thickness maxima and multiple glacial phases. Geology: 36 (5) 411-415
- ↑ Kress, A., et al. 2006. The nature of the transition from lobate debris aprons to lineated valley fill: Mamers Valles, Northern Arabia Terra-Deuteronilus Mensae region on Mars. Lunar. Planet. Sci. 37. Abstract 1323
- ↑ Malin, M., Edgett, K. 2000. Evidence for recent groundwater seepage and surface runoff on Mars. Science 288, 2330–2335.
- ↑ C.M. Dundas, A.S. McEwen, S. Diniega, C.J. Hansen, S. Byrne, J.N. McElwaine. The formation of gullies on Mars today. Geol. Soc. London Spec. Publ., 467 (2019), pp. 67-94, 10.1144/SP467.5
- ↑ C.M. Dundas, S. Diniega, C.J. Hansen, S. Byrne, A.S. McEwen. Seasonal activity and morphological changes in Martian gullies. Icarus, 220 (2012), pp. 124-143, 10.1016/j.icarus.2012.04.005
- ↑ .M. Dundas, S. Diniega, A.S. McEwen. Long-term monitoring of Martian gully formation and evolution with MRO/HiRISE. Icarus, 251 (2015), pp. 244-263, 10.1016/j.icarus.2014.05.013
- ↑ J. Raack, S.J. Conway, T. Heyer, V.T. Bickel, M. Philippe, H. Hiesinger, A. Johnsson, M. Massé. Present-day gully activity in Sisyphi Cavi, Mars - flow-like features and block movements. Icarus, 350 (2020), 10.1016/j.icarus.2020.113899. article #113899
- ↑ S.J. Conway, T. de Haas, T.N. Harrison. Martian gullies: a comprehensive review of observations, mechanisms and insights from Earth analogues. Geol. Soc. London Spec. Publ., 467 (2019), pp. 7-66, 10.1144/SP467.14
- ↑ Dundas, C., et al. 2022. Martian gully activity and the gully sediment transport system. Icarus. (in press)
- ↑ https://www.sciencedirect.com/science/article/pii/S0019103522002408#bb0145
- ↑ https://www.uahirise.org/ESP_057450_1410
- ↑ https://en.wikipedia.org/wiki/Outflow_channels
- ↑ Carr, M.H. (2006), The Surface of Mars. Cambridge Planetary Science Series, Cambridge University Press.
- ↑ Baker, V.R.; Carr, M.H.; Gulick, V.C.; Williams, C.R. & Marley, M.S. "Channels and Valley Networks". In Kieffer, H.H.; Jakosky, B.M.; Snyder, C.W. & Matthews, M.S. Mars. Tucson, AZ: University of Arizona Press.
- ↑ Burr, D.M., McEwan, A.S., and Sakimoto, S.E. (2002). "Recent aqueous floods from the Cerberus Fossae, Mars". Geophys. Res. Lett., 29(1), 10.1029/2001G1013345.
- ↑ ^ Baker, V.R. (1982). The Channels of Mars. Austin: Texas University Press.
- ↑ https://www.uahirise.org/ESP_045833_1845
- ↑ http://hiroc.lpl.arizona.edu/images/PSP/diafotizo.php?ID=PSP_002279_1735
- ↑ https://en.wikipedia.org/wiki/Fossa_(geology)
- ↑ James W. Head; Lionel Wilson; Karl L. Mitchell (2003). "Generation of recent massive water floods at Cerberus Fossae, Mars by dike emplacement, cryospheric cracking, and confined aquifer groundwater release". Geophysical Research Letters. 30 (11): 2265. Bibcode:2003GeoRL..30k..31H. doi:10.1029/2003GL017135
- ↑ Burr, D. et al. 2002. Repeated aqueous flooding from the Cerberus Fossae: evidence for very recently extant deep groundwater on Mars. Icarus. 159: 53-73.
- ↑ https://en.wikipedia.org/wiki/List_of_craters_on_Mars
- ↑ Carr, M.H. (2006) The surface of Mars; Cambridge University Press: Cambridge, UK
- ↑ https://www.space.com/21198-mars-asteroid-strikes-common.html
- ↑ https://www.sciencedirect.com/science/article/abs/pii/S0019103513001693?via%3Dihub
- ↑ Daubar, I., et al. 2013. The current martian cratering rate. Icarus. Volume 225. 506-516.
- ↑ https://www.uahirise.org/hipod/ESP_046876_1465
- ↑ https://www.uahirise.org/hipod/ESP_054486_2210
- ↑ S.J. Kadish, J.W. Head. 2011. Impacts into non-polar ice-rich paleodeposits on Mars: excess ejecta craters, perched craters and pedestal craters as clues to Amazonian climate history. Icarus, 215, pp. 34-46
- ↑ S.J. Kadish, J.W. Head. 2014. The ages of pedestal craters on Mars: evidence for a late Amazonian extended periodic emplacement of decameters-thick mid-latitude ice deposits. Planet. Space Sci., 91, pp. 91-100
- ↑ https://www.uahirise.org/ESP_013514_1630
- ↑ Lefort | first1 = A. | last2 = Russell | first2 = P. | last3 = Thomas | first3 = N. | last4 = McEwen | first4 = A.S. | last5 = Dundas | first5 = C.M. | last6 = Kirk | first6 = R.L. | year = 2009 | title = HiRISE observations of periglacial landforms in Utopia Planitia | url = http://www.agu.org/pubs/crossref/2009/2008JE003264.shtml | journal = Journal of Geophysical Research | volume = 114 | issue = | page = E04005 | doi = 10.1029/2008JE003264 |
- ↑ Morgenstern A, Hauber E, Reiss D, van Gasselt S, Grosse G, Schirrmeister L (2007): Deposition and degradation of a volatile-rich layer in Utopia Planitia, and implications for climate history on Mars. Journal of Geophysical Research: Planets 112, E06010.
- ↑ http://www.uahirise.org/ESP_038821_1235
- ↑ https://www.uahirise.org/hipod/PSP_001938_2265
- ↑ Dundas, C., et al. 2015. Modeling the development of martian sublimation thermokarst landforms. Icarus: 262, 154-169.
- ↑ Dundas | first1 = C. | last2 = Bryrne | first2 = S. | last3 = McEwen | first3 = A. | year = 2015 | title = Modeling the development of martian sublimation thermokarst landforms | url = | journal = Icarus | volume = 262 | issue = | pages = 154–169 | doi=10.1016/j.icarus.2015.07.033
- ↑ Baker, D., J. Head. 2015. Extensive Middle Amazonian mantling of debris aprons and plains in Deuteronilus Mensae, Mars: Implication for the record of mid-latitude glaciation. Icarus: 260, 269-288.
- ↑ Levy, J., J. Head, D. Marchant. 2009. Concentric crater fill in Utopia Planitia: History and interaction between glacial “brain terrain” and periglacial mantle processes. Icarus 202, 462–476.
- ↑ Levy, J., et al. 2009. Concentric crater fill in Utopia Planitia: History and interaction between glacial "brain terrain" and periglacial mantle processes. Icarus: 202, 462-476.
- ↑ https://en.wikipedia.org/wiki/Upper_Plains_Unit
- ↑ Pascuzzo, A., et al. 2019. The formation of irregular polygonal ridge networks, Nili Fossae, Mars: Implications for extensive subsurface channelized fluid flow in the Noachian. Icarus: 319, 852-868.
- ↑ https://www.uahirise.org/hipod/PSP_008189_2080
- ↑ Head, J., J. Mustard. 2006. Breccia dikes and crater-related faults in impact craters on Mars: Erosion and exposure on the floor of a crater 75 km in diameter at the dichotomy boundary, Meteorit. Planet Science: 41, 1675-1690.
- ↑ Moore, J., D. Wilhelms. 2001. Hellas as a possible site of ancient ice-covered lakes on Mars. Icarus: 154, 258-276.
- ↑ Mangold et al. 2007. Mineralogy of the Nili Fossae region with OMEGA/Mars Express data: 2. Aqueous alteration of the crust. J. Geophys. Res., 112, doi:10.1029/2006JE002835.
- ↑ Kerber, L., et al. 2017. Polygonal ridge networks on Mars: Diversity of morphologies and the special case of the Eastern Medusae Fossae Formation. Icarus: 281, 200-219.
- ↑ E. K. Ebinger E., J. Mustard. 2015. LINEAR RIDGES IN THE NILOSYRTIS REGION OF MARS: IMPLICATIONS FOR SUBSURFACE FLUID FLOW. 46th Lunar and Planetary Science Conference (2015) 2034.pdf
- ↑ Saper, L., J. Mustard. 2013. Extensive linear ridge networks in Nili Fossae and Nilosyrtis, Mars: implications for fluid flow in the ancient crust. Geophysical Research letters: 40, 245-249.
- ↑ Kerber L., Schwamb M., Portyankina G. Hansen C. J. Aye K.-M. Global Polygonal Ridge Networks: Evidence for Pervasive Noachian Crustal Groundwater Circulation [#2972]. pdf49th Lunar and Planetary Science Conference 2018 (LPI Contrib. No. 2083). 2972.pdf49th
- ↑ https://news.asu.edu/20220405-citizen-scientists-help-map-ridge-networks-may-hold-records-ancient-groundwater-mars
- ↑ Khuller, A., et al. 2022. Irregular polygonal ridge networks in ancient Noachian terrain on Mars. Icarus. 374. 114833
- ↑ http://adsabs.harvard.edu/abs/1979JGR....84.8147W SAO/NASA ADS Astronomy Abstract Service: Yardangs on Mars
- ↑ http://themis.asu.edu/zoom-20020416a
- ↑ https://www.uahirise.org/ESP_058427_1080
- ↑ http://marsrovers.jpl.nasa.gov/gallery/press/spirit/20070412a.html Mars Exploration Rover Mission: Press Release Images: Spirit. Marsrovers.jpl.nasa.gov
- ↑ https://www.uahirise.org/ESP_061787_2140
- ↑ http://hirise.lpl.arizona.edu/PSP_005383_1255
- ↑ https://www.uahirise.org/ESP_042201_1715
- ↑ https://scholarworks.boisestate.edu/cgi/viewcontent.cgi?article=1207&context=physics_facpubs
- ↑ Jackson, Brian; Lorenz, Ralph; and Davis, Karan. (2018). "A Framework for Relating the Structures and Recovery Statistics in Pressure Time-Series Surveys for Dust Devils". Icarus, 299, 166-174. http://dx.doi.org/10.1016/j.icarus.2017.07.027
- ↑ https://www.jpl.nasa.gov/news/nasas-perseverance-studies-the-wild-winds-of-jezero-crater?utm_source=iContact&utm_medium=email&utm_campaign=nasajpl&utm_content=Day%20in%20Review%20-%206-1-22
- ↑ https://www.science.org/doi/10.1126/sciadv.abn3783
- ↑ Newman, C., et al. 2022. The dynamic atmospheric and aeolian environment of Jezero crater, Mars. Science Advances. Vol. 8. Number 21
- ↑ https://www.jpl.nasa.gov/images/pia26074-martian-whirlwind-takes-the-thorofare
- ↑ Chuang, F.C.; Beyer, R.A.; Bridges, N.T. (2010). Modification of Martian Slope Streaks by Eolian Processes. Icarus, 205 154–164.
- ↑ Heyer, T., et al. 2019. Seasonal formation rates of martian slope streaks. Icarus
- ↑ Schorghofer, N.; Aharonson, O.; Khatiwala, S. 2002. Slope Streaks on Mars: Correlations with Surface Properties and the Potential Role of Water. Geophys. Res. Lett., 29(23), 2126.
- ↑ Sullivan, R. et al. 2001. Mass Movement Slope Streaks Imaged by the Mars Orbiter Camera. J. Geophys. Res., 106(E10), 23,607–23,633.
- ↑ https://agupubs.onlinelibrary.wiley.com/doi/10.1029/2021JE006988
- ↑ Lange, S., et al. 2022. Gardening of the Martian Regolith by Diurnal CO2 Frost and the Formation of Slope Streaks. JGR Planets. Volume127, Issue4. e2021JE006988
- ↑ https://en.wikipedia.org/wiki/Volcanology_of_Mars
- ↑ Head, J.W. 2007. The Geology of Mars: New Insights and Outstanding Questions in The Geology of Mars: Evidence from Earth-Based Analogs, Chapman, M., Ed; Cambridge University Press: Cambridge UK
- ↑ Carr, Michael H. (1973). "Volcanism on Mars". Journal of Geophysical Research. 78 (20): 4049–4062.
- ↑ Barlow, N.G. 2008. Mars: An Introduction to Its Interior, Surface, and Atmosphere; Cambridge University Press: Cambridge, UK
- ↑ https://www.uahirise.org/ESP_057978_1875
- ↑ Wheatley, D., et al., 2019. Clastic pipes and mud volcanism across Mars: Terrestrial analog evidence of past Martian groundwater and subsurface fluid mobilization. Icarus. In Press
- ↑ https://hirise.lpl.arizona.edu/ESP_055307_2215
- ↑ https://www-sciencedirect-com.wikipedialibrary.idm.oclc.org/science/article/pii/S0019103523000507
- ↑ Czechowski, L., et al. 2023. The formation of cone chains in the Chryse Planitia region on Mars and the thermodynamic aspects of this process. Icarus: Volume 396, 15 May 2023, 115473
- ↑ Bernhardt, H.; et al. (2016). "The honeycomb terrain on the Hellas basin floor, mars: a case for salt or ice diapirism: hellas honeycombs as salt/ice diapirs". J. Geophys. Res. 121: 714–738.
- ↑ Weiss, D., J. Head. 2017. HYDROLOGY OF THE HELLAS BASIN AND THE EARLY MARS CLIMATE: WAS THE HONEYCOMB TERRAIN FORMED BY SALT OR ICE DIAPIRISM? Lunar and Planetary Science XLVIII. 1060.pdf
- ↑ Weiss, D.; Head, J. (2017). "Salt or ice diapirism origin for the honeycomb terrain in Hellas basin, Mars?: Implications for the early martian climate". Icarus. 284: 249–263.
- ↑ Carr, M. 2001. Mars Global Surveyor observations of martian fretted terrain. J. Geophys. Res. 106, 23571-23593.
- ↑ Baker, D., J. Head. 2015. Extensive Middle Amazonian mantling of debris aprons and plains in Deuteronilus Mensae, Mars: Implication for the record of mid-latitude glaciation. Icarus: 260, 269-288
- ↑ Kreslavsky, M., J. Head, J. 2002. Mars: Nature and evolution of young, latitude-dependent water-ice-rich mantle. Geophys. Res. Lett. 29, doi:10.1029/ 2002GL015392.
- ↑ https://archive.org/details/PLAN-PIA06808
- ↑ https://www.uahirise.org/PSP_001374_1805
- ↑ Thomas,P., M. Malin, P. James, B. Cantor, R. Williams, P. Gierasch South polar residual cap of Mars: features, stratigraphy, and changes Icarus, 174 (2 SPEC. ISS.). 2005. pp. 535–559. http://doi.org/10.1016/j.icarus.2004.07.028
- ↑ Thomas, P., P. James, W. Calvin, R. Haberle, M. Malin. 2009. Residual south polar cap of Mars: stratigraphy, history, and implications of recent changes Icarus: 203, 352–375 http://doi.org/10.1016/j.icarus.2009.05.014
- ↑ Thomas, P., W.Calvin, P. Gierasch, R. Haberle, P. James, S. Sholes. 2013. Time scales of erosion and deposition recorded in the residual south polar cap of mars Icarus: 225: 923–932 http://doi.org/10.1016/j.icarus.2012.08.038
- ↑ Thomas, P., W. Calvin, B. Cantor, R. Haberle, P. James, S. Lee. 2016. Mass balance of Mars’ residual south polar cap from CTX images and other data Icarus: 268, 118–130 http://doi.org/10.1016/j.icarus.2015.12.038
- ↑ Buhler, Peter, Andrew Ingersoll, Bethany Ehlmann, Caleb Fassett, James Head. 2017. How the martian residual south polar cap develops quasi-circular and heart-shaped pits, troughs, and moats. Icarus: 286, 69-9.
- ↑ Portyankina, G., et al. 2019. How Martian araneiforms get their shapes: morphological analysis and diffusion-limited aggregation model for polar surface erosion Icarus. https://doi.org/10.1016/j.icarus.2019.02.032
- ↑ Kieffer H, Christensen P, Titus T. 2006 Aug 17. CO2 jets formed by sublimation beneath translucent slab ice in Mars' seasonal south polar ice cap. Nature: 442(7104):793-6.
- ↑ https://mars.nasa.gov/resources/possible-development-stages-of-martian-spiders/
- ↑ http://themis.asu.edu/news/gas-jets-spawn-dark-spiders-and-spots-mars-icecap
- ↑ http://spaceref.com/mars/how-gas-carves-channels-on-mars.html
- ↑ https://www.nature.com/articles/s41598-021-82763-7.pdf
- ↑ McKeown, L., et al. 2021. The formation of araneiforms by carbon dioxide venting and vigorous sublimation dynamics under martian atmospheric pressure. Scientific Reports.
- ↑ https://www.livescience.com/spiders-on-mars-explained-dry-ice.html?utm_source=Selligent&utm_medium=email&utm_campaign=LVS_newsletter&utm_content=LVS_newsletter+&utm_term=2946561
- ↑ https://www.uahirise.org/ESP_047247_1150
- ↑ https://www.uahirise.org/ESP_066782_1110
- ↑ https://repository.si.edu/bitstream/handle/10088/19366/nasm_201048.pdf?sequence=1&isAllowed=y
- ↑ Delamere, W., et al. 2010. Color imaging of Mars by the High Resolution Imaging Science Experiment (HiRISE). Icarus. 205 pp. 38–52
- ↑ McEwen, A., et al. 2017. Mars The Prestine Beauty of the Red Planet. University of Arizona Press. Tucson
See Also
*Dust devils *Glaciers on Mars *High Resolution Imaging Science Experiment (HiRISE) *How living on Mars will be different than living on Earth *Layers on Mars *Martian features that are signs of water ice *Martian gullies *Sublimation *Sublimation landscapes on Mars *WaterFurther reading
* Lorenz, R. 2014. The Dune Whisperers. The Planetary Report: 34, 1, 8-14 * Lorenz, R., J. Zimbelman. 2014. Dune Worlds: How Windblown Sand Shapes Planetary Landscapes. Springer Praxis Books / Geophysical Sciences.External links
*Layered Sediments in Hellas Planitia *Changes in dust devil tracks *before and after pictures of a new impact *[https://static.uahirise.org/images/2020/details/cut/ESP_063204_1800.gif Looking for Slope Streaks-old and new pictures of streaks- Features of Mars with HiRISE under HiWish program Shows nearly all major features discovered on Mars. This would be good for teachers covering Mars.
- Martian Ice - Jim Secosky - 16th Annual International Mars Society Convention
- Martian Geology - Jim Secosky - 16th Annual International Mars Society Convention
- Walks on Mars - Jim Secosky - 16th Annual International Mars Society Convention
- https://www.youtube.com/watch?v=kpnTh3qlObk[T. Gordon Wasilewski - Water on Mars - 20th Annual International Mars Society Convention] Describes how to get water from ice in the ground
- Jeffrey Plaut - Subsurface Ice - 21st Annual International Mars Society Convention