Difference between revisions of "Margaritifer Sinus quadrangle"
Suitupshowup (talk | contribs) |
|||
(69 intermediate revisions by 3 users not shown) | |||
Line 1: | Line 1: | ||
− | + | {{Mars atlas}} | |
+ | {| class="wikitable sortable" | ||
+ | |MC-19 | ||
+ | |[[Margaritifer Sinus quadrangle|Margaritifer Sinus]] | ||
+ | |0–30° S | ||
+ | |0–45° W | ||
+ | |[[Mars Quadrangles|Quadrangles]] | ||
+ | |[[Mars Atlas|Atlas]] | ||
+ | |} | ||
− | + | Article written by Jim Secosky. Jim is a retired science teacher who has used the Hubble Space Telescope, the Mars Global Surveyor, and HiRISE.<gallery widths="400" heights="300"> | |
+ | File:Margaritifer Sinus.png | ||
+ | File:PIA00179-MC-19-MargaritiferSinusRegion-19980605.jpg | ||
+ | </gallery> | ||
+ | |||
+ | The '''Margaritifer Sinus quadrangle '' is one of a series of 30 quadrangle maps of Mars used by theUnited States Geological Survey (USGS). The Margaritifer Sinus quadrangle is also referred to as MC-19 (Mars Chart-19).<ref>[http://adsabs.harvard.edu/abs/1992mars.conf..321D Davies, M.E.; Batson, R.M.; Wu, S.S.C. "Geodesy and Cartography" in Kieffer, H.H.; Jakosky, B.M.; Snyder, C.W.; Matthews, M.S., Eds. ''Mars.'' University of Arizona Press: Tucson, 1992.]</ref> The Margaritifer Sinus quadrangle covers the area from 0° to 45° west longitude (O-315 E) and 0° to 30° south latitude. Margaritifer Sinus quadrangle contains Margaritifer Terra and parts of Xanthe Terra, Noachis Terra, Arabia Terra, and Meridiani Planum. | ||
+ | In this article, some of the best pictures from a number of spacecraft will show what the landscape looks like in this region. The origins and significance of all features will be explained as they are currently understood. | ||
− | + | The name of this quadrangle means "pearl bay" after the pearl coast at Cape Comorin in South India.<ref>Blunck, J. 1982. Mars and its Satellites. Exposition Press. Smithtown, N.Y.</ref> | |
+ | This quadrangle shows many signs of past water with evidence of lakes, deltas, ancient rivers, inverted channels, and chaos regions that released water.<ref>Grotzinger, J. and R. Milliken (eds.) 2012. Sedimentary Geology of Mars. SEPM</ref> Margaritifer Sinus contains some of the longest lake-chain systems on Mars, perhaps because of a wetter climate, more groundwater, or some of each factor. The Samara/Himera lake-chain system is about 180 km long, and the Parara/Loire valley network and lake-chain system is about 1100km in length.<ref>[http://linkinghub.elsevier.com/retrieve/pii/S0019103508002728 Fassett, C. and J. Head III. 2008. Valley network-fed, open-basin lakes on Mars: Distribution and implications for Noachian surface and subsurface hydrology. Icarus: 198. 39-56.</ref> A low area between Parana Valle and Loire Vallis is believed to have once held a lake.<ref>[http://linkinghub.elsevier.com/retrieve/pii/S0019103500964650 Goldspiel, J. and S. Squyres. 2000. Groundwater sapping and valley formation on Mars. Icarus. 89: 176-192. </ref> <ref name="Carr2006">Michael H. Carr|title=The surface of Mars|url=https://books.google.com/books?id=uLHlJ6sjohwC|accessdate=21 March 2011|date=2006|publisher=Cambridge University Press|isbn=978-0-521-87201-0</ref> The 154 km diameter Holden Crater also once held a lake.<ref>Cabrol, N. and E. Grin (eds.). 2010. Lakes on Mars. Elsevier. NY.</ref> Near Holden Crater is a graben, called Erythraea Fossa, that once held a chain of three lakes.<ref>Buhler, P. et al. 2011. Evidence for paleolakes in Erythracea Fossa, Mars: Implications for an ancient hydrological cycle. Icarus. 213: 104-115.</ref> | ||
+ | This region contains abundant clay-bearing sediments of Noachian age. It is believed that clay only forms in near-neutral pH water. This is significant because life is probably more likely to form under neutral pH conditions.<ref>Thomas, R., et al. 2017. EXTENSIVE EXPOSURE OF CLAY-BEARING NOACHIAN TERRAIN IN MARGARITIFER TERRA, MARS. Lunar and Planetary Science XLVIII. 2017. 1180.pdf</ref> Spectral studies with CRISM, aboard the Mars Reconnaissance Orbiter, identified the clay as a Fe/Mg-phyllosilicates. This finding is important because biological materials can be preserved in clay. The clay was not mixed with sulfates which form under acid conditions. This region of Mars is famous because the Opportunity Rover landed there on January 25, 2004 at 1.94°S and 354.47°E (5.53° W). Russia's Mars 6 crash landed in Margaritifer Sinus quadrangle at 23.9 S and 19.42 W (340.58 E). | ||
+ | ==Images== | ||
− | [[File: | + | [[File:MOLA opportunity.jpg|thumb|400px|center|Location of Opportunity Rover on surface of Mars.]] |
+ | ==Rock and mineral discoveries at Meridiani Planum== | ||
− | + | [[File:Blueberries eagle.jpg|thumb|left|"Blueberries" (hematite spheres) on a rocky outcrop at Eagle Crater. Note the merged triplet in the upper left.]] | |
+ | Observations from Opportunity Rover showed that the soil at Meridiani Planum was like the soil both at Gusev crater and at Ares Vallis; however, in many places at Meridiani the soil was covered with round, hard, gray spherules that were named "blueberries.” The soil at Gusev was examined by the Spirit Rover, while Pathfinder studied the soil at Ares Vallis. <ref>Yen, A., et al. 2005. An integrated view of the chemistry and mineralogy of martian soils. Nature. 435.: 49-54.</ref> Blueberries were found to be composed almost entirely of the mineral hematite. Scientists concluded that the blueberries generated the spectra signal spotted from orbit by Mars Odyssey. That signal was what prompted researchers to target this site for the landing. Mars Odyssey's data indicated the presence of the mineral hematite which is often produced with the aid of water. Further study showed that the blueberries were concretions formed in the ground by water. They were made by minerals being deposited in the ground from ground water that carried hematite in solution.<ref>Bell, J (ed.) The Martian Surface. 2008. Cambridge University Press. </ref> Eventually, the concretions weathered out from what was overlying rock, and then became concentrated on the surface as a lag deposit. As little as one meter of rock could have produced the observed blueberry covering .<ref>Squyres, S. et al. 2004. The Opportunity Rover's Athena Science Investigation at Meridiani Planum, Mars. Science: 1698-1703.</ref> <ref>Soderblom, L., et al. 2004. Soils of Eagle Crater]and Meridiani Planum at the Opportunity Rover Landing Site. Science: 306. 1723-1726.</ref> Most of the soil consisted of olivine basalt sands that did not come from the local rocks. The sand was probably transported from somewhere else.<ref>Christensen, P., et al. Mineralogy at Meridiani Planum from the Mini-TES Experiment on the Opportunity Rover. Science: 306. 1733-1739.</ref> Olivine is a common mineral found in many volcanic rocks. | ||
+ | <gallery class="center" widths="350px" heights="250px"> | ||
− | + | File:Meridianiblueberriesdrawing.jpg|Drawing showing how "blueberries" came to cover much of surface in Meridiani Planum. | |
− | |||
− | |||
− | |||
− | |||
− | |||
− | |||
− | |||
− | |||
− | |||
− | |||
− | |||
− | |||
</gallery> | </gallery> | ||
− | |||
− | |||
− | |||
− | |||
− | |||
− | |||
===Minerals in dust=== | ===Minerals in dust=== | ||
+ | |||
A Mössbauer spectrum was made of the dust that gathered on Opportunity's capture magnet. The results suggested that the magnetic component of the dust was titanomagnetite, rather than just plain magnetite, as was previously supposed. The spectrum also detected olivine which was interpreted as evidence of a long arid period. If there is moisture available, olivine breaks down. On the other hand, a small amount of hematite in the dust meant that there may have been liquid water for a short time in the early history of the planet.<ref>Goetz, W., et al. 2005. Indication of drier periods on Mars from the chemistry and mineralogy of atmospheric dust. Nature: 436.62-65.</ref> One of the magnets was designed to prevent all magnetic dust from landing on it. Because no dust landed on it, scientists concluded that all dust in the atmosphere is magnetic.<ref>Goetz, W., et al. 2005. Indication of drier periods on mars from the chemistry and mineralogy of atmospheric dust. Nature: 436, 62-65.</ref> | A Mössbauer spectrum was made of the dust that gathered on Opportunity's capture magnet. The results suggested that the magnetic component of the dust was titanomagnetite, rather than just plain magnetite, as was previously supposed. The spectrum also detected olivine which was interpreted as evidence of a long arid period. If there is moisture available, olivine breaks down. On the other hand, a small amount of hematite in the dust meant that there may have been liquid water for a short time in the early history of the planet.<ref>Goetz, W., et al. 2005. Indication of drier periods on Mars from the chemistry and mineralogy of atmospheric dust. Nature: 436.62-65.</ref> One of the magnets was designed to prevent all magnetic dust from landing on it. Because no dust landed on it, scientists concluded that all dust in the atmosphere is magnetic.<ref>Goetz, W., et al. 2005. Indication of drier periods on mars from the chemistry and mineralogy of atmospheric dust. Nature: 436, 62-65.</ref> | ||
Mini-TES was able to get a spectrum of a bright wind streak. The spectrum was a match for bright low-inertia dust that has been seen from orbit. <ref>Bandfield, J., et al. 2003. Spectroscopic identification of carbonate minerals in the Martian dust. Science: 301, 1084-1087.</ref> This information is consistent with the idea that bright wind streaks form from the deposition of airborne dust in the quiet air behind obstacles.<ref>Christensen, P., et al. 2004. Mineralogy at meridian Planum from the Mini-TES Experiment on the Opportunity Rover. Science: 306, 1733.</ref> | Mini-TES was able to get a spectrum of a bright wind streak. The spectrum was a match for bright low-inertia dust that has been seen from orbit. <ref>Bandfield, J., et al. 2003. Spectroscopic identification of carbonate minerals in the Martian dust. Science: 301, 1084-1087.</ref> This information is consistent with the idea that bright wind streaks form from the deposition of airborne dust in the quiet air behind obstacles.<ref>Christensen, P., et al. 2004. Mineralogy at meridian Planum from the Mini-TES Experiment on the Opportunity Rover. Science: 306, 1733.</ref> | ||
+ | |||
===Bedrock minerals=== | ===Bedrock minerals=== | ||
− | Few rocks were visible on the surface where Opportunity landed, but bedrock that was exposed in craters was examined by the suit of instruments on the Rover.<ref>Bell, J., et al. 2004. Pancam Multispectral Imaging Results from the Opportunity Rover at Meridiani Planum. Science: 306.1703-1708.</ref> Because the Rock Abrasion Tool (RAT) found it easy to grind into the bedrocks, it is thought that the rocks are much softer than the rocks at Gusev crater. Bedrock rocks were typified as sedimentary rocks with a high concentration of sulfur in the form of calcium and magnesium sulfates. The sulfates could be kieserite, sulfate anhydrate, bassanite, hexahydrite, epsomite, | + | |
+ | Few rocks were visible on the surface where Opportunity landed, but bedrock that was exposed in craters was examined by the suit of instruments on the Rover.<ref>Bell, J., et al. 2004. Pancam Multispectral Imaging Results from the Opportunity Rover at Meridiani Planum. Science: 306.1703-1708.</ref> Because the Rock Abrasion Tool (RAT) found it easy to grind into the bedrocks, it is thought that the rocks are much softer than the rocks at Gusev crater. Bedrock rocks were typified as sedimentary rocks with a high concentration of sulfur in the form of calcium and magnesium sulfates. The sulfates could be kieserite, sulfate anhydrate, bassanite, hexahydrite, epsomite, or gypsum. Salts, such as halite, bischofite, antarcticite, bloedite, vanthoffite, or gluberite may also be present.<ref>Christensen, P., et al. 2004 Mineralogy at Meridiani Planum from the Mini-TES Experiment on the Opportunity Rover. Science: 306. 1733-1739.</ref> <ref>Squyres, S. et al. 2004. In Situ Evidence for an Ancient Aqueous Environment at Meridian Planum, Mars. Science: 306. 1709-1714.</ref> | ||
+ | |||
[[File:Sol1167B P2419 L257atc br2victoriacrater.jpg|thumb|left|Close view of Victoria Crater The material at the top consists of loose, jumbled rock, then a bit further down into the crater, abruptly transitions to solid bedrock. This transition point is marked by a bright band of rock, visible around the entire crater.]] | [[File:Sol1167B P2419 L257atc br2victoriacrater.jpg|thumb|left|Close view of Victoria Crater The material at the top consists of loose, jumbled rock, then a bit further down into the crater, abruptly transitions to solid bedrock. This transition point is marked by a bright band of rock, visible around the entire crater.]] | ||
− | The rocks containing sulfates are light-toned, as compared to rocks and rocks examined by landers/rovers at other locations on Mars. The spectra of these light toned rocks, containing hydrated sulfates, were similar to spectra taken by the Thermal Emission Spectrometer on board the Mars Global Surveyor. Water may have existed over a very wide area because the same spectrum, as observed from orbiting instruments, covers a large area.<ref>Hynek, B. 2004. Implications for hydrologic processes on Mars from extensive bedrock outcrops throughout Terra Meridiani. Nature: 431. 156-159.</ref> | + | |
+ | The rocks containing sulfates are light-toned, as compared to rocks and rocks examined by landers/rovers at other locations on Mars. The spectra of these light-toned rocks, containing hydrated sulfates, were similar to spectra taken by the Thermal Emission Spectrometer on board the Mars Global Surveyor. Water may have existed over a very wide area because the same spectrum, as observed from orbiting instruments, covers a large area.<ref>Hynek, B. 2004. Implications for hydrologic processes on Mars from extensive bedrock outcrops throughout Terra Meridiani. Nature: 431. 156-159.</ref> | ||
The Alpha Particle X-ray Spectrometer (APXS) found rather high levels of phosphorus in the rocks. Similar high levels were found by other rovers at Ares Vallis and Gusev Crater; thus, it has been hypothesized that the mantle of Mars may be phosphorus-rich.<ref>Dreibus,G. and H. Wanke. 1987. Volatiles on Earth and Mars: a comparison. Icarus. 71:225-240</ref> Minerals in the rocks could have originated by acid weathering of basalt. If there were acid-rich fluids, it would be more difficult for life to have started here. <ref>Squyers, S., et al. 2004. In Situ Evidence for an Ancient Aqueous Environment at Meridiani Planum Mars. Science: 306, 1709-1714.</ref> Because the solubility of phosphorus is related to the solubility of uranium, thorium, and rare earth elements, they are all also expected to be enriched in rocks.<ref>Rieder, R., et al. 2004. Chemistry of Rocks and Soils at Meridiani Planum from the Alpha Particle X-ray Spectrometer. Science. 306. 1746-1749</ref> | The Alpha Particle X-ray Spectrometer (APXS) found rather high levels of phosphorus in the rocks. Similar high levels were found by other rovers at Ares Vallis and Gusev Crater; thus, it has been hypothesized that the mantle of Mars may be phosphorus-rich.<ref>Dreibus,G. and H. Wanke. 1987. Volatiles on Earth and Mars: a comparison. Icarus. 71:225-240</ref> Minerals in the rocks could have originated by acid weathering of basalt. If there were acid-rich fluids, it would be more difficult for life to have started here. <ref>Squyers, S., et al. 2004. In Situ Evidence for an Ancient Aqueous Environment at Meridiani Planum Mars. Science: 306, 1709-1714.</ref> Because the solubility of phosphorus is related to the solubility of uranium, thorium, and rare earth elements, they are all also expected to be enriched in rocks.<ref>Rieder, R., et al. 2004. Chemistry of Rocks and Soils at Meridiani Planum from the Alpha Particle X-ray Spectrometer. Science. 306. 1746-1749</ref> | ||
− | [[File:PIA19109-MarsOpportunityRover-EndeavourCrater-CapeTribulation-20150122.jpg |right|thumb|320px| Cape Tribulation at Endeavour Crater ]] | + | [[File:PIA19109-MarsOpportunityRover-EndeavourCrater-CapeTribulation-20150122.jpg |right|thumb|320px| Cape Tribulation at Endeavour Crater]] |
+ | |||
On the rim of Endeavour crater, Opportunity Rover found a white vein that was later categorized as being pure gypsum.<ref>http://www.nasa.gov/mission_pages/mer/news/mer20111207.html</ref> <ref>https://www.sciencedaily.com/releases/2012/01/120125093619.htm</ref> This vein was formed when water carrying gypsum in solution deposited the mineral in a crack in the rock. A picture of this vein, called "Homestake" formation, is shown below. Often there are minerals dissolved in water, if the water evaporates the minerals will appear. | On the rim of Endeavour crater, Opportunity Rover found a white vein that was later categorized as being pure gypsum.<ref>http://www.nasa.gov/mission_pages/mer/news/mer20111207.html</ref> <ref>https://www.sciencedaily.com/releases/2012/01/120125093619.htm</ref> This vein was formed when water carrying gypsum in solution deposited the mineral in a crack in the rock. A picture of this vein, called "Homestake" formation, is shown below. Often there are minerals dissolved in water, if the water evaporates the minerals will appear. | ||
− | <gallery class="center" widths=" | + | |
+ | <gallery class="center" widths="380px" heights="360px"> | ||
+ | |||
File:PIA15033 Pancam sol2769 L456atc brvein.jpg|Vein full of gypsum Water with dissolved gypsum deposited the mineral in a crack. | File:PIA15033 Pancam sol2769 L456atc brvein.jpg|Vein full of gypsum Water with dissolved gypsum deposited the mineral in a crack. | ||
</gallery> | </gallery> | ||
+ | |||
===Evidence for water=== | ===Evidence for water=== | ||
+ | |||
[[File:LastChance D JG03-B058R1 br.jpg|thumb|left|Cross-bedding features in rock "Last Chance". Marks are the interpretations of cross-lamination patterns presented that suggest an origin under flowing water. Images taken by the microscopic imager on NASA's Opportunity rover. The microscopic view is a mosaic of some of the 152 microscopic imager frames.]] | [[File:LastChance D JG03-B058R1 br.jpg|thumb|left|Cross-bedding features in rock "Last Chance". Marks are the interpretations of cross-lamination patterns presented that suggest an origin under flowing water. Images taken by the microscopic imager on NASA's Opportunity rover. The microscopic view is a mosaic of some of the 152 microscopic imager frames.]] | ||
− | Examination of Meridiani rocks revealed strong support for past water on Mars in this location. For instance, the mineral called jarosite which only forms in water was found in all bedrocks. This discovery proved that water once existed in Meridiani Planum<ref>Klingelhofer, G. et al. 2004. Jarosite and Hematite at Meridiani Planum from Opportunity's Mossbauer Spectrometer. Science: 306. 1740-1745.</ref> In addition, some rocks showed small laminations (layers) with shapes that are only made by gently flowing water.<ref>Herkenhoff, K., et al. 2004. Evidence from Opportunity's Microscopic Imager for Water on Meridian Planum. Science: 306. 1727-1730</ref> The first such laminations were found in a rock called "The Dells."<ref> Squyres, S. et al. 2004. In Situ Evidence for an Ancient Aqueous Environment at Meridian Planum, Mars. Science: 306. 1709-1714.</ref> A picture of cross-stratification, also called cross-bedding, is shown on the left. | + | |
− | Box-shaped holes in some rocks were caused by sulfates forming large crystals. When these sulfate crystals later dissolved, holes, called vugs, were left behind.<ref>Herkenhoff, K., et al. 2004. Evidence from Opportunity's Microscopic Imager for Water on Meridian Planum. Science: 306. 1727-1730</ref> | + | Examination of Meridiani rocks revealed strong support for past water on Mars in this location. For instance, the mineral called jarosite which only forms in water was found in all bedrocks. This discovery proved that water once existed in Meridiani Planum<ref>Klingelhofer, G. et al. 2004. Jarosite and Hematite at Meridiani Planum from Opportunity's Mossbauer Spectrometer. Science: 306. 1740-1745.</ref> In addition, some rocks showed small laminations (layers) with shapes that are only made by gently flowing water.<ref>Herkenhoff, K., et al. 2004. Evidence from Opportunity's Microscopic Imager for Water on Meridian Planum. Science: 306. 1727-1730</ref> The first such laminations were found in a rock called "The Dells."<ref>Squyres, S. et al. 2004. In Situ Evidence for an Ancient Aqueous Environment at Meridian Planum, Mars. Science: 306. 1709-1714.</ref> A picture of cross-stratification, also called cross-bedding, is shown on the left. |
− | [[File:Voids on bedrock on Mars.jpg |right|thumb|320px| Voids or "vugs" inside the rock These formed when crystals dissolved. ]] | + | Box-shaped holes in some rocks were caused by sulfates forming large crystals. When these sulfate crystals later dissolved, holes, called vugs, were left behind.<ref>Herkenhoff, K., et al. 2004. Evidence from Opportunity's Microscopic Imager for Water on Meridian Planum. Science: 306. 1727-1730</ref> |
− | The concentration of the element bromine in rocks was highly variable probably because it is very soluble. Water may have concentrated it in places before it evaporated. <ref>Yen, A., et al. 2005. An integrated view of the chemistry and mineralogy of martian soils. Nature. 435.: 49-54.</ref> | + | |
+ | [[File:Voids on bedrock on Mars.jpg |right|thumb|320px| Voids or "vugs" inside the rock These formed when crystals dissolved.]] | ||
+ | |||
+ | The concentration of the element bromine in rocks was highly variable probably because it is very soluble. Water may have concentrated it in places before it evaporated. <ref>Yen, A., et al. 2005. An integrated view of the chemistry and mineralogy of martian soils. Nature. 435.: 49-54.</ref> | ||
+ | |||
===Rock from impact=== | ===Rock from impact=== | ||
+ | |||
One rock, "Bounce Rock," found sitting on the sandy plains was found to be ejecta from an impact crater. Its chemistry was different than the bedrocks. Comprising mostly pyroxene and plagioclase and no olivine, it closely resembled a part, Lithology B, of the shergottite meteorite EETA 79001, a meteorite known to have come from Mars. Bounce rock received its name by being near an airbag bounce mark.<ref>Squyres, S. et al. 2004. In Situ Evidence for an Ancient Aqueous Environment at Meridian Planum, Mars. Science: 306. 1709-1714.</ref> Since its chemical makeup was different than other rocks, researchers believe that it may have been thrown out by an impact some 75 Km away. Its weak nature is also consistent with an impact origin. Bounce Rock may be the type of rock existing several kilometers beneath the surface.<ref>Squyres, S., et al. 2004. The Opportunity Rover’s Athena Science Investigation at Meridiani Planum, Mars. Science: 306, 1698-1703.</ref> Impacts are very useful to scientists since they can provide samples from deep in the ground. | One rock, "Bounce Rock," found sitting on the sandy plains was found to be ejecta from an impact crater. Its chemistry was different than the bedrocks. Comprising mostly pyroxene and plagioclase and no olivine, it closely resembled a part, Lithology B, of the shergottite meteorite EETA 79001, a meteorite known to have come from Mars. Bounce rock received its name by being near an airbag bounce mark.<ref>Squyres, S. et al. 2004. In Situ Evidence for an Ancient Aqueous Environment at Meridian Planum, Mars. Science: 306. 1709-1714.</ref> Since its chemical makeup was different than other rocks, researchers believe that it may have been thrown out by an impact some 75 Km away. Its weak nature is also consistent with an impact origin. Bounce Rock may be the type of rock existing several kilometers beneath the surface.<ref>Squyres, S., et al. 2004. The Opportunity Rover’s Athena Science Investigation at Meridiani Planum, Mars. Science: 306, 1698-1703.</ref> Impacts are very useful to scientists since they can provide samples from deep in the ground. | ||
+ | |||
===Meteorites=== | ===Meteorites=== | ||
+ | |||
Opportunity Rover found meteorites just sitting on the plains. The first one analyzed with Opportunity's instruments was called "Heatshield Rock," as it was found near where Opportunity's headshield landed. Examination with the Miniature Thermal Emission Spectrometer (Mini-TES), Mossbauer spectrometer, and APXS lead researchers to state that it was similar to part of the Shergottite meteorite that is assumed to be from Mars.<ref>Squyers, S., et al. 2004. The Opportunity Rover’s Athena Science Investigation at Meridiani Planum, Mars. Science: 306, 1698-1709.</ref> <ref>McSween, et al. 1994. Meteoritics:29, 757.</ref> <ref>Christensen, P., et al. 2004. Mineralogy at Meridani Planum from the Mini-TES Experiment on the Opportunity Rover. Science: 306, 1733-1739.</ref> The APXS determined it was composed of 93% iron and 7% nickel. The cobble named "Fig Tree Barberton" is thought to be a stony or stony-iron meteorite (mesosiderite silicate), <ref>Squyres, S., et al. 2009. Exploration of Victoria Crater by the Mars Rover Opportunity. Science: 1058-1061.</ref> <ref>Schroder,C., et al. 2008. J. Geophys. Res.: 113.</ref> while "Allan Hills," and "Zhong Shan" may be iron meteorites. | Opportunity Rover found meteorites just sitting on the plains. The first one analyzed with Opportunity's instruments was called "Heatshield Rock," as it was found near where Opportunity's headshield landed. Examination with the Miniature Thermal Emission Spectrometer (Mini-TES), Mossbauer spectrometer, and APXS lead researchers to state that it was similar to part of the Shergottite meteorite that is assumed to be from Mars.<ref>Squyers, S., et al. 2004. The Opportunity Rover’s Athena Science Investigation at Meridiani Planum, Mars. Science: 306, 1698-1709.</ref> <ref>McSween, et al. 1994. Meteoritics:29, 757.</ref> <ref>Christensen, P., et al. 2004. Mineralogy at Meridani Planum from the Mini-TES Experiment on the Opportunity Rover. Science: 306, 1733-1739.</ref> The APXS determined it was composed of 93% iron and 7% nickel. The cobble named "Fig Tree Barberton" is thought to be a stony or stony-iron meteorite (mesosiderite silicate), <ref>Squyres, S., et al. 2009. Exploration of Victoria Crater by the Mars Rover Opportunity. Science: 1058-1061.</ref> <ref>Schroder,C., et al. 2008. J. Geophys. Res.: 113.</ref> while "Allan Hills," and "Zhong Shan" may be iron meteorites. | ||
+ | |||
[[Image: Meteoritemars.jpg |thumb|200px|left|Meteorite found on Mars by Opportunity Rover in the Margaritifer Sinus quadrangle]] | [[Image: Meteoritemars.jpg |thumb|200px|left|Meteorite found on Mars by Opportunity Rover in the Margaritifer Sinus quadrangle]] | ||
+ | |||
===Geological history=== | ===Geological history=== | ||
− | Observations at the site have led scientists to believe that the area was flooded with water a number of times but also dried out several times. <ref>Squyres, S. et al. 2004. In Situ Evidence for an Ancient Aqueous Environment at Meridian Planum, Mars. Science: 306. 1709-1714.</ref> As water came and went, sulfates were deposited. After sulfates cemented the sediments, hematite concretions grew by precipitation from groundwater. Some sulfates formed into large crystals which later dissolved to leave vugs which are fancy names for holes. Several lines of evidence point toward an arid climate in the past billion years or so, but a climate supporting water, at least for a time, in the distant past.<ref>Clark, B. et al. Chemistry and mineralogy of outcrops at Meridiani Planum. Earth Planet. Sci. Lett. 240: 73-94.</ref> <ref>Salvatore, M., M. Kraft1, C. Edwards, P. Christensen. 2015. Geologic History of margaritifer Basin, Mars: Evidence for a Prolonged Yet Episodic Hydrologic System. 46th Lunar and Planetary Science Conference (2015) 1463.pdf</ref> Moreover, the high albedo (light toned) materials near the landing site appear to be widespread; hence, a large region may have had a watery past.<ref>Squyres, S. et al. 2004. In Situ Evidence for an Ancient Aqueous Environment at Meridian Planum, Mars. Science: 306. 1709-1714.</ref> | + | |
+ | Observations at the site have led scientists to believe that the area was flooded with water a number of times but also dried out several times. <ref>Squyres, S. et al. 2004. In Situ Evidence for an Ancient Aqueous Environment at Meridian Planum, Mars. Science: 306. 1709-1714.</ref> As water came and went, sulfates were deposited. After sulfates cemented the sediments, hematite concretions grew by precipitation from groundwater. Precipitation happens when minerals come out of solution. Some sulfates formed into large crystals which later dissolved to leave vugs which are fancy names for holes. Several lines of evidence point toward an arid climate in the past billion years or so, but a climate supporting water, at least for a time, in the distant past.<ref>Clark, B. et al. Chemistry and mineralogy of outcrops at Meridiani Planum. Earth Planet. Sci. Lett. 240: 73-94.</ref> <ref>Salvatore, M., M. Kraft1, C. Edwards, P. Christensen. 2015. Geologic History of margaritifer Basin, Mars: Evidence for a Prolonged Yet Episodic Hydrologic System. 46th Lunar and Planetary Science Conference (2015) 1463.pdf</ref> Moreover, the high albedo (light toned) materials near the landing site appear to be widespread; hence, a large region may have had a watery past.<ref>Squyres, S. et al. 2004. In Situ Evidence for an Ancient Aqueous Environment at Meridian Planum, Mars. Science: 306. 1709-1714.</ref> | ||
[[File:Pia17271 sol3355-rearhaz 3botanybaysolanderpoint.jpg|600pxr|Botany Bay and Solander Point (in the distance)]] | [[File:Pia17271 sol3355-rearhaz 3botanybaysolanderpoint.jpg|600pxr|Botany Bay and Solander Point (in the distance)]] | ||
− | |||
Line 73: | Line 94: | ||
==Vallis== | ==Vallis== | ||
+ | |||
'''''Vallis''''' (plural ''valles'') is the Latin word for ''valley''. It is used in planetary geology for the naming of valley landform features on other planets. | '''''Vallis''''' (plural ''valles'') is the Latin word for ''valley''. It is used in planetary geology for the naming of valley landform features on other planets. | ||
− | Vallis was used for old river valleys that were discovered on Mars, when probes were first sent to Mars. The Viking Orbiters caused a revolution in our ideas about water on Mars; huge river valleys were found in many areas. Space craft cameras showed that floods of water broke through dams, carved deep valleys, eroded grooves into bedrock, and traveled thousands of kilometers.<ref >=Hugh H. Kieffer|title=Mars|url=https://books.google.com/books?id=NoDvAAAAMAAJ|accessdate=7 March 2011|date=1992|publisher=University of Arizona Press|</ref><ref >Raeburn, P. 1998. ''Uncovering the Secrets of the Red Planet Mars''. National Geographic Society. Washington, D.C.</ref> <ref name="Moore1990">Moore, P. et al. 1990. '' The Atlas of the Solar System''. Mitchell Beazley Publishers, New York.</ref> | + | Vallis was used for old river valleys that were discovered on Mars, when probes were first sent to Mars. The Viking Orbiters caused a revolution in our ideas about water on Mars; huge river valleys were found in many areas. Space craft cameras showed that floods of water broke through dams, carved deep valleys, eroded grooves into bedrock, and traveled thousands of kilometers.<ref><nowiki>=Hugh H. Kieffer|title=Mars|url=</nowiki>https://books.google.com/books?id=NoDvAAAAMAAJ|accessdate=7 March 2011|date=1992|publisher=University of Arizona Press|</ref><ref>Raeburn, P. 1998. ''Uncovering the Secrets of the Red Planet Mars''. National Geographic Society. Washington, D.C.</ref> <ref name="Moore1990">Moore, P. et al. 1990. '' The Atlas of the Solar System''. Mitchell Beazley Publishers, New York.</ref> |
− | Nirgal Vallis is a tributary of Uzboi Vallis. Nirgal Vallis is believed to have formed by groundwater sapping, not by precipitation. Sapping is the process in which groundwater undermines a slope and causes it to collapse and weather backward.<ref> https://www.britannica.com/science/sapping</ref> Many, but not all, channels on Mars seem to start with sapping. So, there could be rivers on Mars that started this way even it is too cold for water to flow. If water came out of the ground | + | Nirgal Vallis is a tributary of Uzboi Vallis. Nirgal Vallis is believed to have formed by groundwater sapping, not by precipitation. Sapping is the process in which groundwater undermines a slope and causes it to collapse and weather backward.<ref>https://www.britannica.com/science/sapping</ref> Many, but not all, channels on Mars seem to start with sapping. So, there could be rivers on Mars that started this way even it is too cold for water to flow. If water came out of the ground by sapping on Mars, the top would freeze, but water could continue to flow under ice for a time. The flowing water would be able to produce many (but not all) of the channels that we observe today. |
Spectral analyses have found phyllosilicates (clays) that are iron-magnesium smectites.<ref>Buczkowski D. et al. 2010. LPS XLI Abstract #1458.</ref> <ref>Buczkowski D. et al. 2013. LPS XLIV Abstract #2331.</ref> Some researchers believe these were formed by interaction with groundwater. Over a wide area, Al-smectites are found on top of Fe/Mg smectites.<ref>Buczkowski, D., K. Seelos, C. Beck, and S. Murchie. 2015. POTENTIAL ALTERATION BY GROUNDWATER FLOW IN NW NOACHIS TERRA: GEOMORPHIC AND MINERALOGIC EVIDENCE IN NIRGAL AND HER DESHER VALLES. 46th Lunar and Planetary Science Conference 2271.pdf</ref> | Spectral analyses have found phyllosilicates (clays) that are iron-magnesium smectites.<ref>Buczkowski D. et al. 2010. LPS XLI Abstract #1458.</ref> <ref>Buczkowski D. et al. 2013. LPS XLIV Abstract #2331.</ref> Some researchers believe these were formed by interaction with groundwater. Over a wide area, Al-smectites are found on top of Fe/Mg smectites.<ref>Buczkowski, D., K. Seelos, C. Beck, and S. Murchie. 2015. POTENTIAL ALTERATION BY GROUNDWATER FLOW IN NW NOACHIS TERRA: GEOMORPHIC AND MINERALOGIC EVIDENCE IN NIRGAL AND HER DESHER VALLES. 46th Lunar and Planetary Science Conference 2271.pdf</ref> | ||
− | Smectite clays, also called Montmorillonites, are clays that are usually formed from igneous materials, especially volcanic ash. They have three layers and can easily absorb water. Their occurrence on Mars supports the idea that Mars was once wet.<ref> Sorrell, C. Rocks and Minerals. 1973. Golden Press. N.Y.</ref> | + | Smectite clays, also called Montmorillonites, are clays that are usually formed from igneous materials, especially volcanic ash. They have three layers and can easily absorb water. Their occurrence on Mars supports the idea that Mars was once wet.<ref>Sorrell, C. Rocks and Minerals. 1973. Golden Press. N.Y.</ref> |
− | + | [[File:Mapjonesmartopo.jpg|600pxr|Map showing locations of several valleys in the Margaritifer Sinus quadrangle: Landon Valles, Nirgal Vallis, Uzboi Vallis, Arda Valles, Samara Valles, Himera Valles, and Clota Vallis]] | |
− | File:Mapjonesmartopo.jpg|Map showing locations of several valleys in the Margaritifer Sinus quadrangle: Landon Valles, Nirgal Vallis, Uzboi Vallis, Arda Valles, Samara Valles, Himera Valles, and Clota Vallis | + | |
− | < | + | |
+ | Map showing locations of several valleys in the Margaritifer Sinus quadrangle: Landon Valles, Nirgal Vallis, Uzboi Vallis, Arda Valles, Samara Valles, Himera Valles, and Clota Vallis | ||
+ | |||
+ | <gallery class="center" widths="380px" heights="360px"> | ||
+ | |||
+ | File:Ladon Valles.JPG|Ladon Valles, as seen by HiRISE. | ||
− | |||
− | |||
− | |||
File:Nirgal Valliswide.jpg|The long channel Nirgal Vallis is shown where it connects to Uzboi Vallis. The crater Luki is 21 km in diameter. Picture taken by THEMIS. | File:Nirgal Valliswide.jpg|The long channel Nirgal Vallis is shown where it connects to Uzboi Vallis. The crater Luki is 21 km in diameter. Picture taken by THEMIS. | ||
File:Nirgal Vallis Close-up.JPG|Nirgal Vallis Close-up, as seen by THEMIS | File:Nirgal Vallis Close-up.JPG|Nirgal Vallis Close-up, as seen by THEMIS | ||
− | File:ESP 048068 1545channel.jpg|Channel that enters Kasimov Crater, as seen by HiRISE under HiWish program | + | File:ESP 048068 1545channel.jpg|Channel that enters Kasimov Crater, as seen by HiRISE under [[HiWish program]] |
</gallery> | </gallery> | ||
− | == Aureum Chaos == | + | ==Aureum Chaos== |
+ | |||
+ | [[File:24807whitebuttei.jpg|left|thumb|320px|Enlargement of white butte, as seen by HiRISE under HiWish program Box shows size of a football field.]] | ||
+ | |||
Aureum Chaos is a major canyon system and collapsed area. It is probably a major source of water for large outflow channels. | Aureum Chaos is a major canyon system and collapsed area. It is probably a major source of water for large outflow channels. | ||
− | Large outflow channels on Mars are believed to be caused by catastrophic discharges of ground water. Many of the channels begin in chaotic terrain, where the ground has apparently collapsed. In the collapsed section, blocks of undisturbed material can be seen. The OMEGA experiment on Mars Express discovered clay minerals (phyllosilicates) in a variety of places in Aureum Chaos. | + | Large outflow channels on Mars are believed to be caused by catastrophic discharges of ground water. Many of the channels begin in chaotic terrain, where the ground has apparently collapsed. In the collapsed section, blocks of undisturbed material can be seen. The OMEGA experiment on Mars Express discovered clay minerals (phyllosilicates) in a variety of places in Aureum Chaos. Since clay minerals need water to form, the area may once have contained large amounts of water.<ref>[http://hirise.lpl.arizona.edu/PSP_0040261765 (HiRISE image; Observation ID: PSP_0040261765)]</ref> Scientists are interested in determining what parts of Mars contained water because evidence of past or present life may be found there. |
+ | |||
+ | [[File:ESP 028618 1760layers.jpg|right|thumb|320px|Layered butte in Aureum Chaos]] | ||
+ | |||
+ | <gallery class="center" widths="380px" heights="360px"> | ||
− | |||
File:Canyons and Mesas of Aureum Chaos in Oxia Palus.JPG|Huge canyons in Aureum Chaos. Gullies are rare at this latitude. Picture taken by THEMIS. | File:Canyons and Mesas of Aureum Chaos in Oxia Palus.JPG|Huge canyons in Aureum Chaos. Gullies are rare at this latitude. Picture taken by THEMIS. | ||
File:Aureum Chaos from Themis.JPG|Aureum Chaos, as seen from THEMIS. | File:Aureum Chaos from Themis.JPG|Aureum Chaos, as seen from THEMIS. | ||
− | + | ||
− | + | ||
</gallery> | </gallery> | ||
==Layers== | ==Layers== | ||
+ | |||
+ | [[File:45075 1715layersclose.jpg|right|thumb|320px|Close view of layers in wall of Aurorae Chaos, as seen by HiRISE under HiWish program Box shows the size of football field.]] | ||
+ | |||
Many places on Mars show rocks arranged in layers. Rock can form layers in a variety of ways. Volcanoes, wind, or water can produce layers.<ref>http://hirise.lpl.arizona.edu?PSP_008437_1750 |title=HiRISE | High Resolution Imaging Science Experiment |publisher=Hirise.lpl.arizona.edu?psp_008437_1750 </ref> | Many places on Mars show rocks arranged in layers. Rock can form layers in a variety of ways. Volcanoes, wind, or water can produce layers.<ref>http://hirise.lpl.arizona.edu?PSP_008437_1750 |title=HiRISE | High Resolution Imaging Science Experiment |publisher=Hirise.lpl.arizona.edu?psp_008437_1750 </ref> | ||
− | A detailed discussion of layering with many Martian examples can be found in Sedimentary Geology of Mars.<ref >Grotzinger, J. and R. Milliken (eds.). 2012. Sedimentary Geology of Mars. SEPM.</ref> | + | A detailed discussion of layering with many Martian examples can be found in Sedimentary Geology of Mars.<ref>Grotzinger, J. and R. Milliken (eds.). 2012. Sedimentary Geology of Mars. SEPM.</ref> |
Sometimes the layers are of different colors. Light-toned rocks on Mars have been associated with hydrated minerals like sulfates.<ref>Weitz, C. et al. 2017. LIGHT-TONED MATERIALS OF MELAS CHASMA: EVIDENCE FOR THEIR FORMATION ON MARS. Lunar and Planetary Science XLVIII (2017) 2794.pdf</ref><ref>Weitz C., et al. 2015. Icarus: 251: 291-314</ref><ref>Weitz, C. 2016. Journal of Geophysical Research: Planets, 2016, 121(5): 805-835.</ref><ref>Bishop, J., et al. 2013. What the ancient phyllosilicates at Mawrth Vallis can tell us about possible habitability on early Mars. Planetary and Space Science: 86, 130-149.</ref> The Mars Rover[[Opportunity]] examined such layers close up with several instruments. Some layers are probably made up of fine particles because they seem to break up into fine dust. Other layers break up into large boulders so they are probably much harder. Basalt, a volcanic rock, is thought to in the layers that form boulders. Basalt has been identified on Mars in many places. Instruments on orbiting spacecraft have detected clay (also called phyllosilicate) in some layers. | Sometimes the layers are of different colors. Light-toned rocks on Mars have been associated with hydrated minerals like sulfates.<ref>Weitz, C. et al. 2017. LIGHT-TONED MATERIALS OF MELAS CHASMA: EVIDENCE FOR THEIR FORMATION ON MARS. Lunar and Planetary Science XLVIII (2017) 2794.pdf</ref><ref>Weitz C., et al. 2015. Icarus: 251: 291-314</ref><ref>Weitz, C. 2016. Journal of Geophysical Research: Planets, 2016, 121(5): 805-835.</ref><ref>Bishop, J., et al. 2013. What the ancient phyllosilicates at Mawrth Vallis can tell us about possible habitability on early Mars. Planetary and Space Science: 86, 130-149.</ref> The Mars Rover[[Opportunity]] examined such layers close up with several instruments. Some layers are probably made up of fine particles because they seem to break up into fine dust. Other layers break up into large boulders so they are probably much harder. Basalt, a volcanic rock, is thought to in the layers that form boulders. Basalt has been identified on Mars in many places. Instruments on orbiting spacecraft have detected clay (also called phyllosilicate) in some layers. | ||
Layers can be hardened by the action of groundwater. Martian ground water probably moved hundreds of kilometers, and in the process it dissolved many minerals from the rock it passed through. When ground water surfaces in low areas containing sediments, water evaporates in the thin atmosphere and leaves behind minerals as deposits and/or cementing agents. Consequently, layers of dust could not later easily erode away since they were cemented together. | Layers can be hardened by the action of groundwater. Martian ground water probably moved hundreds of kilometers, and in the process it dissolved many minerals from the rock it passed through. When ground water surfaces in low areas containing sediments, water evaporates in the thin atmosphere and leaves behind minerals as deposits and/or cementing agents. Consequently, layers of dust could not later easily erode away since they were cemented together. | ||
− | <gallery class="center" widths=" | + | |
+ | <gallery class="center" widths="380px" heights="360px"> | ||
+ | |||
File:ESP 042055 1580layers.jpg|Layers exposed in a pit in Lotto Crater, as seen by HiRISE under HiWish program The light-toned layers may contain sulfates which are good for preserving traces of ancient life. | File:ESP 042055 1580layers.jpg|Layers exposed in a pit in Lotto Crater, as seen by HiRISE under HiWish program The light-toned layers may contain sulfates which are good for preserving traces of ancient life. | ||
File:042055 1580layerswide.jpg|Enlargement of previous image of layers, as seen by HiRISE under HiWish program | File:042055 1580layerswide.jpg|Enlargement of previous image of layers, as seen by HiRISE under HiWish program | ||
− | + | ||
</gallery> | </gallery> | ||
− | <gallery class="center" widths=" | + | |
+ | <gallery class="center" widths="380px" heights="360px"> | ||
File:47633 1765layersclose.jpg|Close view of layers and light-toned material Light-toned material is often a sign of past water. | File:47633 1765layersclose.jpg|Close view of layers and light-toned material Light-toned material is often a sign of past water. | ||
− | File:47633 1765layerswide.jpg|Close view of layers, as seen by HiRISE under | + | File:47633 1765layerswide.jpg|Close view of layers |
+ | |||
+ | File:Layers in mounds ESP 037545 1730 02.jpg|Layers in mounds, as seen by HiRISE under HiWish program | ||
</gallery> | </gallery> | ||
− | == Mars Science Laboratory == | + | ==Mars Science Laboratory== |
− | The Margaritifer Sinus quadrangle is an interesting location of geologists, as several sites in the Margaritifer Sinus quadrangle have been proposed as areas to send NASA's Mars rover, the Mars Science Laboratory. Both Holden Crater and Eberswalde Crater made the cut to be among the top four.<ref>[http://www.space.com/8598-mars-rover-landing-site-narrowed-4-choices.html Next Mars Rover's Landing Site Narrowed to 4 Choices. JR Minkel, 15 June 2010 (SPACE.com)]</ref> Miyamoto Crater, also in this quadrangle, was in the top 7 sites chosen. Holden Crater is believed to have once been a lake. Actually, it is now believed that it held two lakes.<ref>Grant,J., et al. 2008. HiRISE imaging of impact megabreccia and sub-meter aqueous strata in Holden Crater, Mars. Geology. 36: 195-198.</ref> The first was longer lived and was formed from drainage within the crater and precipitation. The last lake began when water dammed up in Uzboi Vallis broke through a divide, then rapidly drained into Holden Crater. Because there are rocks meters in diameter on the crater floor, it is thought | + | The Margaritifer Sinus quadrangle is an interesting location of geologists, as several sites in the Margaritifer Sinus quadrangle have been proposed as areas to send NASA's Mars rover, the Mars Science Laboratory. Both Holden Crater and Eberswalde Crater made the cut to be among the top four.<ref>[http://www.space.com/8598-mars-rover-landing-site-narrowed-4-choices.html Next Mars Rover's Landing Site Narrowed to 4 Choices. JR Minkel, 15 June 2010 (SPACE.com)]</ref> Miyamoto Crater, also in this quadrangle, was in the top 7 sites chosen. Holden Crater is believed to have once been a lake. Actually, it is now believed that it held two lakes.<ref>Grant,J., et al. 2008. HiRISE imaging of impact megabreccia and sub-meter aqueous strata in Holden Crater, Mars. Geology. 36: 195-198.</ref> The first was longer lived and was formed from drainage within the crater and precipitation. The last lake began when water dammed up in Uzboi Vallis broke through a divide, then rapidly drained into Holden Crater. Because there are rocks meters in diameter on the crater floor, it is thought there was a powerful flood when water flowed into the crater.<ref>Cabrol, N. and E. Grin (eds.). 2010. Lakes on Mars. Elsevier. NY.</ref> |
− | <gallery class="center" widths="200px" heights="300px" > | + | <gallery class="center" widths="200px" heights="300px"> |
File:Holden Crater Close-up.JPG|Close-up of Channels on Rim of Holden Crater, as seen by THEMIS. Click on image to see more details. | File:Holden Crater Close-up.JPG|Close-up of Channels on Rim of Holden Crater, as seen by THEMIS. Click on image to see more details. | ||
</gallery> | </gallery> | ||
− | Eberswalde Crater contains a delta | + | Eberswalde Crater contains a delta.<ref>[http://dsc.discovery.com/news/2008/11/21/mars-landing-sites-02.html NASA Narrows List of Next Mars Landing Sites. Irene Klotz, 21 November 2008. (Discovery News)] https://web.archive.org/web/20090225074621/http://dsc.discovery.com/news/2008/11/21/mars-landing-sites-02.html </ref> There is a great deal of evidence that Miyamoto Crater once contained rivers and lakes. Many minerals, such as clays, chlorides, sulfates, and iron oxides, have been discovered there.<ref>[http://www.planetary.brown.edu/pdfs/3964.pdf Murchie, S. et al. 2009. A synthesis of Martian aqueous mineralogy after 1 Mars year of observations from the Mars Reconnaissance Orbiter. Journal of Geophysical Research: 114.] {{doi|10.1029/2009JE003342}}</ref> |
− | These minerals are often formed in water. A picture below shows an inverted channel in Miyamoto Crater. Inverted channels formed from accumulated sediments that were cemented by minerals. These channels eroded into the surface, and then the whole area was covered over with sediments. When the sediments were later eroded away, the place where the river channel existed remained because the hardened material that was deposited in the channel was resistant to erosion.<ref>[http://hirise.lpl.arizona.edu/ HiRISE - High Resolution Imaging Science Experiment]</ref> Iani Chaos, pictured below, was among the top 33 landing sites. Deposits of hematite and gypsum have been found there.<ref >"Iani_Chaos">[http://themis.mars.asu.edu/feature/31 The Floods of Iani Chaos (Mars Odyssey THEMIS)]</ref> Those minerals are usually formed in connection with water. | + | These minerals are often formed in water. A picture below shows an inverted channel in Miyamoto Crater. Inverted channels formed from accumulated sediments that were cemented by minerals. These channels eroded into the surface, and then the whole area was covered over with sediments. When the sediments were later eroded away, the place where the river channel existed remained because the hardened material that was deposited in the channel was resistant to erosion.<ref>[http://hirise.lpl.arizona.edu/ HiRISE - High Resolution Imaging Science Experiment]</ref> Iani Chaos, pictured below, was among the top 33 landing sites. Deposits of hematite and gypsum have been found there.<ref>"Iani_Chaos">[http://themis.mars.asu.edu/feature/31 The Floods of Iani Chaos (Mars Odyssey THEMIS)]</ref> Those minerals are usually formed in connection with water. |
+ | |||
+ | [[File:Iani Chaos.JPG|thumb|400px|left|Iani Chaos, as seen by THEMIS. Sand from eroding mesas is covering brighter floor material. Click on image to see relationship of Iani Chaos to other local features.]] | ||
+ | |||
+ | [[File:Landing zone in Iani Chaos.JPG|thumb|400px|right|Possible future landing zone in Iani Chaos, as seen by THEMIS.]] | ||
+ | |||
− | + | The aim of the Mars Science Laboratory, later named [[Curiosity]], is to search for signs of ancient life. It is hoped that a later mission could then return samples from sites that the Mars Science Laboratory identified as probably containing remains of life. To safely bring the craft down, a 12-mile-wide, smooth, flat circle must be found. Geologists hope to examine places where water once ponded.<ref>http://themis.mars.asu.edu/feature/31</ref> They would like to examine sediment layers. In the end, it was decided to send the Mars science Laboratory, called "Curiosity," to Gale Crater in the Aeolis quadrangle." | |
− | |||
− | |||
− | </ | ||
− | + | ==Inverted relief== | |
− | |||
Some places on Mars show inverted relief. In these locations, a stream bed may be a raised feature, instead of a valley. The inverted former stream channels may be caused by the deposition of large rocks or due to cementation. In either case erosion would erode the surrounding land and leave the old channel as a raised ridge because the ridge will be more resistant to erosion. An image below, taken with HiRISE of Miyamoto Crater displays a ridge that is an old channel that has become inverted.<ref>[http://hiroc.lpl.arizona.edu/images/PSP/diafotizo.php?ID=PSP_002279_1735 Sinuous Ridges Near Aeolis Mensae (HiRISE image; Observation ID: PSP_002279_1735)]</ref> | Some places on Mars show inverted relief. In these locations, a stream bed may be a raised feature, instead of a valley. The inverted former stream channels may be caused by the deposition of large rocks or due to cementation. In either case erosion would erode the surrounding land and leave the old channel as a raised ridge because the ridge will be more resistant to erosion. An image below, taken with HiRISE of Miyamoto Crater displays a ridge that is an old channel that has become inverted.<ref>[http://hiroc.lpl.arizona.edu/images/PSP/diafotizo.php?ID=PSP_002279_1735 Sinuous Ridges Near Aeolis Mensae (HiRISE image; Observation ID: PSP_002279_1735)]</ref> | ||
− | <gallery class="center" widths=" | + | <gallery class="center" widths="380px" heights="360px"> |
File:Miyamoto Crater.JPG| Inverted Channel in Miyamoto Crater The scale bar is 500 meters long. | File:Miyamoto Crater.JPG| Inverted Channel in Miyamoto Crater The scale bar is 500 meters long. | ||
</gallery> | </gallery> | ||
− | == Deltas == | + | |
− | Researchers have found a number of examples of deltas that formed in Martian lakes. | + | ==Deltas== |
− | <gallery class="center" widths=" | + | |
− | File:Delta in Margaritifer Sinus.jpg|Delta in Margaritifer Sinus quadrangle as seen by THEMIS. | + | [[File:26126contextb22 018333 1548delta.jpg|left|thumb|400px|Delta on Holden Crater floor, as seen by CTX|Wide view of a delta in Holden Crater, as seen by CTX]] |
− | File:Distributary fan-delta.jpg|Probable delta in Eberswalde Crater that lies to the NE of Holden Crater, as seen by Mars Global Surveyor | + | |
− | + | ||
+ | Researchers have found a number of examples of deltas that formed in Martian lakes. Deltas are major signs that Mars once had a lot of water. Deltas often require deep water over a long period of time to form. Also, the water level needs to be stable to keep sediment from washing away. Deltas have been found over a wide geographical range. Below, are pictures of a few.<ref>[http://www.geo.brown.edu/geocourses/geo292/papers/Irwin2005-JGR-Lakes2005JE002460.pdf Irwin III, R. et al. 2005. An intense terminal epoch of widespread fluvial activity on early Mars: 2. Increased runoff and paleolake development. Journal of Geophysical Research: 10. E12S15]</ref> | ||
+ | |||
+ | <gallery class="center" widths="380px" heights="360px"> | ||
+ | |||
+ | File:Delta in Margaritifer Sinus.jpg|Delta in Margaritifer Sinus quadrangle, as seen by THEMIS. | ||
+ | File:Distributary fan-delta.jpg|Probable delta in Eberswalde Crater that lies to the NE of Holden Crater, as seen by Mars Global Surveyor. | ||
+ | |||
</gallery> | </gallery> | ||
+ | |||
==Craters== | ==Craters== | ||
− | Impact craters generally have a rim with ejecta around them | + | |
− | <ref > Hugh H. Kieffer (1992). Mars. University of Arizona Press. ISBN 978-0-8165-1257-7.</ref> Sometimes craters will display layers. Craters can show us what lies deep under the surface. | + | [[File:Wikijones.jpg|600pxr|Jones Crater, as seen by CTX camera (on Mars Reconnaissance Orbiter). Regions on the floor containing layers, fans, and dunes are labeled.]] |
− | In December 2011, Opportunity Rover discovered a vein of gypsum sticking out of the soil along the rim of Endeavour crater. Tests confirmed that it contained calcium, sulfur, and water. The mineral gypsum is the best match for the data. It likely formed from mineral rich water moving through a crack in the rock. The vein, called "Homestake," is in Mars' Meridiani plain. It could have been produced in conditions more neutral than the harshly acidic conditions indicated by the other sulfate deposits; hence this environment may have been more hospitable for a large variety of living organisms.<ref>http://www.nasa.gov/home/hqnews/2011/dec/HQ_11-403_Mars_Rover_Gypsum.html</ref> | + | |
− | <gallery class="center" widths=" | + | |
− | File:Wikivinogradovfans.jpg|Fans on crater floor on the rim of Vinogradov Crater, as seen by CTX camera (on Mars Reconnaissance Orbiter). | + | Jones Crater, as seen by CTX camera (on Mars Reconnaissance Orbiter). Regions on the floor containing layers, fans, and dunes are labeled. |
− | + | ||
− | File:ESP 056784 1520doublecrater.jpg|Double crater | + | |
+ | Impact craters generally have a rim with ejecta around them; in contrast, volcanic craters usually do not have a rim or ejecta deposits. As craters get larger (greater than 10 km in diameter), they usually have a central peak.<ref>[http://www.lpi.usra.edu/publications/slidesets/stones/ Stones, Wind, and Ice: A Guide to Martian Impact Craters. Compiled by Nadine G. Barlow, Virgil L. Sharpton]</ref> The peak is caused by a rebound of the crater floor following the impact. | ||
+ | <ref>Hugh H. Kieffer (1992). Mars. University of Arizona Press. ISBN 978-0-8165-1257-7.</ref> Sometimes craters will display layers. Craters can show us what lies deep under the surface. | ||
+ | In December 2011, Opportunity Rover discovered a vein of gypsum sticking out of the soil along the rim of Endeavour crater. Tests confirmed that it contained calcium, sulfur, and water. The mineral gypsum is the best match for the data. It likely formed from mineral rich water moving through a crack in the rock. The vein, called "Homestake," is in Mars' Meridiani plain. It could have been produced in conditions more neutral than the harshly acidic conditions indicated by the other sulfate deposits; hence, this environment may have been more hospitable for a large variety of living organisms.<ref>http://www.nasa.gov/home/hqnews/2011/dec/HQ_11-403_Mars_Rover_Gypsum.html</ref> | ||
+ | |||
+ | <gallery class="center" widths="380px" heights="360px"> | ||
+ | |||
+ | File:Wikivinogradovfans.jpg|Fans on crater floor on the rim of Vinogradov Crater, as seen by CTX camera (on Mars Reconnaissance Orbiter). | ||
+ | |||
+ | File:ESP 056784 1520doublecrater.jpg|Double crater The impactor may have broken apart right before striking the surface. | ||
</gallery> | </gallery> | ||
− | ==Unnamed | + | |
+ | ==Unnamed Channels== | ||
+ | |||
There is enormous evidence that water once flowed in river valleys on Mars.<ref>Baker, V., et al. 2015. Fluvial geomorphology on Earth-like planetary surfaces: a review. Geomorphology. 245, 149–182.</ref> <ref>Carr, M. 1996. in Water on Mars. Oxford Univ. Press.</ref> Images of curved channels have been seen in images from Mars spacecraft dating back to the early seventies with the [[Mariner 9]] orbiter.<ref>Baker, V. 1982. The Channels of Mars. Univ. of Tex. Press, Austin, TX</ref> <ref>Baker, V., R. Strom, R., V. Gulick, J. Kargel, G. Komatsu, V. Kale. 1991. Ancient oceans, ice sheets and the hydrological cycle on Mars. Nature 352, 589–594.</ref> <ref>Carr, M. 1979. Formation of Martian flood features by release of water from confined aquifers. J. Geophys. Res. 84, 2995–300.</ref> <ref>Komar, P. 1979. Comparisons of the hydraulics of water flows in Martian outflow channels with flows of similar scale on Earth. Icarus 37, 156–181.</ref> A study published in June 2017, calculated that the volume of water needed to carve all the channels on Mars was even larger than the proposed ocean that the planet may have had. Water was probably recycled many times from the ocean to rainfall around Mars.<ref>http://spaceref.com/mars/how-much-water-was-needed-to-carve-valleys-on-mars.html</ref><ref>Luo, W., et al. 2017. New Martian valley network volume estimate consistent with ancient ocean and warm and wet climate. Nature Communications 8. Article number: 15766 (2017). doi:10.1038/ncomms15766</ref> | There is enormous evidence that water once flowed in river valleys on Mars.<ref>Baker, V., et al. 2015. Fluvial geomorphology on Earth-like planetary surfaces: a review. Geomorphology. 245, 149–182.</ref> <ref>Carr, M. 1996. in Water on Mars. Oxford Univ. Press.</ref> Images of curved channels have been seen in images from Mars spacecraft dating back to the early seventies with the [[Mariner 9]] orbiter.<ref>Baker, V. 1982. The Channels of Mars. Univ. of Tex. Press, Austin, TX</ref> <ref>Baker, V., R. Strom, R., V. Gulick, J. Kargel, G. Komatsu, V. Kale. 1991. Ancient oceans, ice sheets and the hydrological cycle on Mars. Nature 352, 589–594.</ref> <ref>Carr, M. 1979. Formation of Martian flood features by release of water from confined aquifers. J. Geophys. Res. 84, 2995–300.</ref> <ref>Komar, P. 1979. Comparisons of the hydraulics of water flows in Martian outflow channels with flows of similar scale on Earth. Icarus 37, 156–181.</ref> A study published in June 2017, calculated that the volume of water needed to carve all the channels on Mars was even larger than the proposed ocean that the planet may have had. Water was probably recycled many times from the ocean to rainfall around Mars.<ref>http://spaceref.com/mars/how-much-water-was-needed-to-carve-valleys-on-mars.html</ref><ref>Luo, W., et al. 2017. New Martian valley network volume estimate consistent with ancient ocean and warm and wet climate. Nature Communications 8. Article number: 15766 (2017). doi:10.1038/ncomms15766</ref> | ||
− | <gallery class="center" widths=" | + | <gallery class="center" widths="380px" heights="360px"> |
− | File:ESP 047076 1565channels.jpg|Group of channels on a mound | + | |
− | File:ESP 045800 1540channel.jpg|Channel | + | File:ESP 047076 1565channels.jpg|Group of channels on a mound Arrows show eroded craters. |
− | File:ESP 053211 1495channel.jpg|Channel just west of Uzboi Vallis | + | File:ESP 045800 1540channel.jpg|Channel |
+ | File:ESP 053211 1495channel.jpg|Channel just west of Uzboi Vallis | ||
</gallery> | </gallery> | ||
− | == Other landscapes in Margaritifer Sinus quadrangle == | + | |
− | <gallery class="center" widths=" | + | ==Other landscapes in Margaritifer Sinus quadrangle== |
+ | |||
+ | [[File:46050 1775race.jpg|left|thumb|320p|Mesa, as seen by HiRISE under HiWish program This may make for a good race around a mesa someday in the far future.]] | ||
+ | |||
+ | [[File:55149 1765tarscolor.jpg|right|thumb|320px|Close, color view of unusual transverse aeolian ridges, TAR's, as seen by HiRISE under HiWish program These features may have had variable local winds to make the wavy tops.]] | ||
+ | |||
+ | <gallery class="center" widths="380px" heights="360px"> | ||
File:Wikiarsinoesfanswide.jpg|Light-toned deposit in Arsinoes Chaos, as seen by CTX camera (on Mars Reconnaissance Orbiter). | File:Wikiarsinoesfanswide.jpg|Light-toned deposit in Arsinoes Chaos, as seen by CTX camera (on Mars Reconnaissance Orbiter). | ||
+ | |||
+ | File:Layers and light toned deposits ESP 037545 1730 01.jpg|Light-toned deposit in Arsinoes Chaos, as seen by HiRISE under HiWish program. These kind of deposits often contain water bearing minerals like sulfates. | ||
+ | |||
File:WikiESP 039563 1730yardangs.jpg|Yardangs formed in light-toned material and surrounded by dark, volcanic basalt sand, as seen by HiRISE under HiWish program | File:WikiESP 039563 1730yardangs.jpg|Yardangs formed in light-toned material and surrounded by dark, volcanic basalt sand, as seen by HiRISE under HiWish program | ||
File:WikiESP 039563 1730yardangsclose.jpg|Close-up image of yardangs, as seen by HiRISE under HiWish program. Arrows point to transverse aeolian ridges. TAR's are a type of dune. Note this is an enlargement of the previous image from HiRISE. | File:WikiESP 039563 1730yardangsclose.jpg|Close-up image of yardangs, as seen by HiRISE under HiWish program. Arrows point to transverse aeolian ridges. TAR's are a type of dune. Note this is an enlargement of the previous image from HiRISE. | ||
− | + | File:ESP 045299 1545coloredclose.jpg|Color image of patterned ground, as seen by HiRISE under HiWish program | |
− | |||
− | File:ESP 045299 1545coloredclose.jpg|Color image of patterned ground | ||
</gallery> | </gallery> | ||
− | ==See | + | |
− | *[[ | + | ==See also== |
+ | |||
+ | |||
+ | *[[High Resolution Imaging Science Experiment (HiRISE)]] | ||
+ | *[[HiWish program]] | ||
+ | |||
+ | *[[Layers on Mars]] | ||
+ | *[[Margaritifer Terra]] | ||
+ | *[[Mars Global Surveyor]] | ||
+ | |||
*[[Rivers on Mars]] | *[[Rivers on Mars]] | ||
+ | |||
==References== | ==References== | ||
+ | |||
{{reflist|colwidth=30em}} | {{reflist|colwidth=30em}} | ||
+ | |||
==Recommended reading== | ==Recommended reading== | ||
− | * Hugh H. Kieffer (1992). Mars. University of Arizona Press. ISBN 978-0-8165-1257-7. | + | |
− | * Squyers, S., et al. 2004. The Opportunity Rover’s Athena Science Investigation at Meridiani Planum, Mars. Science: 306, 1698-1709 | + | *Hugh H. Kieffer (1992). Mars. University of Arizona Press. ISBN 978-0-8165-1257-7. |
+ | *Squyers, S., et al. 2004. The Opportunity Rover’s Athena Science Investigation at Meridiani Planum, Mars. Science: 306, 1698-1709 | ||
*[https://www.jpl.nasa.gov/news/press_kits/merlaunch.pdf Opportunity Rover Press Kit] | *[https://www.jpl.nasa.gov/news/press_kits/merlaunch.pdf Opportunity Rover Press Kit] | ||
− | *[https://en.wikipedia.org/wiki/Margaritifer_Sinus_quadrangle | + | *[https://en.wikipedia.org/wiki/Margaritifer_Sinus_quadrangle Margaritifer_Sinus_quadrangle Wikipedia] |
− | == External links == | + | |
− | *[https://www.youtube.com/watch?v=OlElwjpgjoM | + | ==External links== |
− | *[https://www.youtube.com/watch?v=jhkYhmRfsTU | + | |
− | *[https://www.youtube.com/watch?v=daiUW_O6gOM | + | *[https://www.youtube.com/watch?v=OlElwjpgjoM What did NASA's Opportunity Rover find on Mars? (Episode 2)] |
+ | *[https://www.youtube.com/watch?v=jhkYhmRfsTU NASA Extends Recovery Efforts for Opportunity as the Mars Rover Remains Silent After Dust Storm] | ||
+ | *[https://www.youtube.com/watch?v=daiUW_O6gOM Mars Rover Opportunity Landing] | ||
*[https://www.bing.com/videos/search?q=opportunity+rover+launch&view=detail&mid=5C1DA58CF9E7E2748EC25C1DA58CF9E7E2748EC2&FORM=VIRE Mars Exploration Rover Opportunity Launch] | *[https://www.bing.com/videos/search?q=opportunity+rover+launch&view=detail&mid=5C1DA58CF9E7E2748EC25C1DA58CF9E7E2748EC2&FORM=VIRE Mars Exploration Rover Opportunity Launch] | ||
[[Category: Mars Atlas]] | [[Category: Mars Atlas]] |
Latest revision as of 14:46, 24 December 2023
MC-19 | Margaritifer Sinus | 0–30° S | 0–45° W | Quadrangles | Atlas |
Article written by Jim Secosky. Jim is a retired science teacher who has used the Hubble Space Telescope, the Mars Global Surveyor, and HiRISE.
The 'Margaritifer Sinus quadrangle is one of a series of 30 quadrangle maps of Mars used by theUnited States Geological Survey (USGS). The Margaritifer Sinus quadrangle is also referred to as MC-19 (Mars Chart-19).[1] The Margaritifer Sinus quadrangle covers the area from 0° to 45° west longitude (O-315 E) and 0° to 30° south latitude. Margaritifer Sinus quadrangle contains Margaritifer Terra and parts of Xanthe Terra, Noachis Terra, Arabia Terra, and Meridiani Planum.
In this article, some of the best pictures from a number of spacecraft will show what the landscape looks like in this region. The origins and significance of all features will be explained as they are currently understood.
The name of this quadrangle means "pearl bay" after the pearl coast at Cape Comorin in South India.[2] This quadrangle shows many signs of past water with evidence of lakes, deltas, ancient rivers, inverted channels, and chaos regions that released water.[3] Margaritifer Sinus contains some of the longest lake-chain systems on Mars, perhaps because of a wetter climate, more groundwater, or some of each factor. The Samara/Himera lake-chain system is about 180 km long, and the Parara/Loire valley network and lake-chain system is about 1100km in length.[4] A low area between Parana Valle and Loire Vallis is believed to have once held a lake.[5] [6] The 154 km diameter Holden Crater also once held a lake.[7] Near Holden Crater is a graben, called Erythraea Fossa, that once held a chain of three lakes.[8]
This region contains abundant clay-bearing sediments of Noachian age. It is believed that clay only forms in near-neutral pH water. This is significant because life is probably more likely to form under neutral pH conditions.[9] Spectral studies with CRISM, aboard the Mars Reconnaissance Orbiter, identified the clay as a Fe/Mg-phyllosilicates. This finding is important because biological materials can be preserved in clay. The clay was not mixed with sulfates which form under acid conditions. This region of Mars is famous because the Opportunity Rover landed there on January 25, 2004 at 1.94°S and 354.47°E (5.53° W). Russia's Mars 6 crash landed in Margaritifer Sinus quadrangle at 23.9 S and 19.42 W (340.58 E).
Contents
Images
Rock and mineral discoveries at Meridiani Planum
Observations from Opportunity Rover showed that the soil at Meridiani Planum was like the soil both at Gusev crater and at Ares Vallis; however, in many places at Meridiani the soil was covered with round, hard, gray spherules that were named "blueberries.” The soil at Gusev was examined by the Spirit Rover, while Pathfinder studied the soil at Ares Vallis. [10] Blueberries were found to be composed almost entirely of the mineral hematite. Scientists concluded that the blueberries generated the spectra signal spotted from orbit by Mars Odyssey. That signal was what prompted researchers to target this site for the landing. Mars Odyssey's data indicated the presence of the mineral hematite which is often produced with the aid of water. Further study showed that the blueberries were concretions formed in the ground by water. They were made by minerals being deposited in the ground from ground water that carried hematite in solution.[11] Eventually, the concretions weathered out from what was overlying rock, and then became concentrated on the surface as a lag deposit. As little as one meter of rock could have produced the observed blueberry covering .[12] [13] Most of the soil consisted of olivine basalt sands that did not come from the local rocks. The sand was probably transported from somewhere else.[14] Olivine is a common mineral found in many volcanic rocks.
Minerals in dust
A Mössbauer spectrum was made of the dust that gathered on Opportunity's capture magnet. The results suggested that the magnetic component of the dust was titanomagnetite, rather than just plain magnetite, as was previously supposed. The spectrum also detected olivine which was interpreted as evidence of a long arid period. If there is moisture available, olivine breaks down. On the other hand, a small amount of hematite in the dust meant that there may have been liquid water for a short time in the early history of the planet.[15] One of the magnets was designed to prevent all magnetic dust from landing on it. Because no dust landed on it, scientists concluded that all dust in the atmosphere is magnetic.[16] Mini-TES was able to get a spectrum of a bright wind streak. The spectrum was a match for bright low-inertia dust that has been seen from orbit. [17] This information is consistent with the idea that bright wind streaks form from the deposition of airborne dust in the quiet air behind obstacles.[18]
Bedrock minerals
Few rocks were visible on the surface where Opportunity landed, but bedrock that was exposed in craters was examined by the suit of instruments on the Rover.[19] Because the Rock Abrasion Tool (RAT) found it easy to grind into the bedrocks, it is thought that the rocks are much softer than the rocks at Gusev crater. Bedrock rocks were typified as sedimentary rocks with a high concentration of sulfur in the form of calcium and magnesium sulfates. The sulfates could be kieserite, sulfate anhydrate, bassanite, hexahydrite, epsomite, or gypsum. Salts, such as halite, bischofite, antarcticite, bloedite, vanthoffite, or gluberite may also be present.[20] [21]
The rocks containing sulfates are light-toned, as compared to rocks and rocks examined by landers/rovers at other locations on Mars. The spectra of these light-toned rocks, containing hydrated sulfates, were similar to spectra taken by the Thermal Emission Spectrometer on board the Mars Global Surveyor. Water may have existed over a very wide area because the same spectrum, as observed from orbiting instruments, covers a large area.[22] The Alpha Particle X-ray Spectrometer (APXS) found rather high levels of phosphorus in the rocks. Similar high levels were found by other rovers at Ares Vallis and Gusev Crater; thus, it has been hypothesized that the mantle of Mars may be phosphorus-rich.[23] Minerals in the rocks could have originated by acid weathering of basalt. If there were acid-rich fluids, it would be more difficult for life to have started here. [24] Because the solubility of phosphorus is related to the solubility of uranium, thorium, and rare earth elements, they are all also expected to be enriched in rocks.[25]
On the rim of Endeavour crater, Opportunity Rover found a white vein that was later categorized as being pure gypsum.[26] [27] This vein was formed when water carrying gypsum in solution deposited the mineral in a crack in the rock. A picture of this vein, called "Homestake" formation, is shown below. Often there are minerals dissolved in water, if the water evaporates the minerals will appear.
Evidence for water
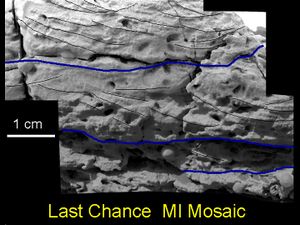
Examination of Meridiani rocks revealed strong support for past water on Mars in this location. For instance, the mineral called jarosite which only forms in water was found in all bedrocks. This discovery proved that water once existed in Meridiani Planum[28] In addition, some rocks showed small laminations (layers) with shapes that are only made by gently flowing water.[29] The first such laminations were found in a rock called "The Dells."[30] A picture of cross-stratification, also called cross-bedding, is shown on the left. Box-shaped holes in some rocks were caused by sulfates forming large crystals. When these sulfate crystals later dissolved, holes, called vugs, were left behind.[31]
The concentration of the element bromine in rocks was highly variable probably because it is very soluble. Water may have concentrated it in places before it evaporated. [32]
Rock from impact
One rock, "Bounce Rock," found sitting on the sandy plains was found to be ejecta from an impact crater. Its chemistry was different than the bedrocks. Comprising mostly pyroxene and plagioclase and no olivine, it closely resembled a part, Lithology B, of the shergottite meteorite EETA 79001, a meteorite known to have come from Mars. Bounce rock received its name by being near an airbag bounce mark.[33] Since its chemical makeup was different than other rocks, researchers believe that it may have been thrown out by an impact some 75 Km away. Its weak nature is also consistent with an impact origin. Bounce Rock may be the type of rock existing several kilometers beneath the surface.[34] Impacts are very useful to scientists since they can provide samples from deep in the ground.
Meteorites
Opportunity Rover found meteorites just sitting on the plains. The first one analyzed with Opportunity's instruments was called "Heatshield Rock," as it was found near where Opportunity's headshield landed. Examination with the Miniature Thermal Emission Spectrometer (Mini-TES), Mossbauer spectrometer, and APXS lead researchers to state that it was similar to part of the Shergottite meteorite that is assumed to be from Mars.[35] [36] [37] The APXS determined it was composed of 93% iron and 7% nickel. The cobble named "Fig Tree Barberton" is thought to be a stony or stony-iron meteorite (mesosiderite silicate), [38] [39] while "Allan Hills," and "Zhong Shan" may be iron meteorites.
Geological history
Observations at the site have led scientists to believe that the area was flooded with water a number of times but also dried out several times. [40] As water came and went, sulfates were deposited. After sulfates cemented the sediments, hematite concretions grew by precipitation from groundwater. Precipitation happens when minerals come out of solution. Some sulfates formed into large crystals which later dissolved to leave vugs which are fancy names for holes. Several lines of evidence point toward an arid climate in the past billion years or so, but a climate supporting water, at least for a time, in the distant past.[41] [42] Moreover, the high albedo (light toned) materials near the landing site appear to be widespread; hence, a large region may have had a watery past.[43]
Botany Bay and Solander Point (in the distance)
Vallis
Vallis (plural valles) is the Latin word for valley. It is used in planetary geology for the naming of valley landform features on other planets. Vallis was used for old river valleys that were discovered on Mars, when probes were first sent to Mars. The Viking Orbiters caused a revolution in our ideas about water on Mars; huge river valleys were found in many areas. Space craft cameras showed that floods of water broke through dams, carved deep valleys, eroded grooves into bedrock, and traveled thousands of kilometers.[44][45] [46] Nirgal Vallis is a tributary of Uzboi Vallis. Nirgal Vallis is believed to have formed by groundwater sapping, not by precipitation. Sapping is the process in which groundwater undermines a slope and causes it to collapse and weather backward.[47] Many, but not all, channels on Mars seem to start with sapping. So, there could be rivers on Mars that started this way even it is too cold for water to flow. If water came out of the ground by sapping on Mars, the top would freeze, but water could continue to flow under ice for a time. The flowing water would be able to produce many (but not all) of the channels that we observe today. Spectral analyses have found phyllosilicates (clays) that are iron-magnesium smectites.[48] [49] Some researchers believe these were formed by interaction with groundwater. Over a wide area, Al-smectites are found on top of Fe/Mg smectites.[50] Smectite clays, also called Montmorillonites, are clays that are usually formed from igneous materials, especially volcanic ash. They have three layers and can easily absorb water. Their occurrence on Mars supports the idea that Mars was once wet.[51]
Map showing locations of several valleys in the Margaritifer Sinus quadrangle: Landon Valles, Nirgal Vallis, Uzboi Vallis, Arda Valles, Samara Valles, Himera Valles, and Clota Vallis
Channel that enters Kasimov Crater, as seen by HiRISE under HiWish program
Aureum Chaos
Aureum Chaos is a major canyon system and collapsed area. It is probably a major source of water for large outflow channels.
Large outflow channels on Mars are believed to be caused by catastrophic discharges of ground water. Many of the channels begin in chaotic terrain, where the ground has apparently collapsed. In the collapsed section, blocks of undisturbed material can be seen. The OMEGA experiment on Mars Express discovered clay minerals (phyllosilicates) in a variety of places in Aureum Chaos. Since clay minerals need water to form, the area may once have contained large amounts of water.[52] Scientists are interested in determining what parts of Mars contained water because evidence of past or present life may be found there.
Layers
Many places on Mars show rocks arranged in layers. Rock can form layers in a variety of ways. Volcanoes, wind, or water can produce layers.[53]
A detailed discussion of layering with many Martian examples can be found in Sedimentary Geology of Mars.[54]
Sometimes the layers are of different colors. Light-toned rocks on Mars have been associated with hydrated minerals like sulfates.[55][56][57][58] The Mars RoverOpportunity examined such layers close up with several instruments. Some layers are probably made up of fine particles because they seem to break up into fine dust. Other layers break up into large boulders so they are probably much harder. Basalt, a volcanic rock, is thought to in the layers that form boulders. Basalt has been identified on Mars in many places. Instruments on orbiting spacecraft have detected clay (also called phyllosilicate) in some layers.
Layers can be hardened by the action of groundwater. Martian ground water probably moved hundreds of kilometers, and in the process it dissolved many minerals from the rock it passed through. When ground water surfaces in low areas containing sediments, water evaporates in the thin atmosphere and leaves behind minerals as deposits and/or cementing agents. Consequently, layers of dust could not later easily erode away since they were cemented together.
Mars Science Laboratory
The Margaritifer Sinus quadrangle is an interesting location of geologists, as several sites in the Margaritifer Sinus quadrangle have been proposed as areas to send NASA's Mars rover, the Mars Science Laboratory. Both Holden Crater and Eberswalde Crater made the cut to be among the top four.[59] Miyamoto Crater, also in this quadrangle, was in the top 7 sites chosen. Holden Crater is believed to have once been a lake. Actually, it is now believed that it held two lakes.[60] The first was longer lived and was formed from drainage within the crater and precipitation. The last lake began when water dammed up in Uzboi Vallis broke through a divide, then rapidly drained into Holden Crater. Because there are rocks meters in diameter on the crater floor, it is thought there was a powerful flood when water flowed into the crater.[61]
Eberswalde Crater contains a delta.[62] There is a great deal of evidence that Miyamoto Crater once contained rivers and lakes. Many minerals, such as clays, chlorides, sulfates, and iron oxides, have been discovered there.[63] These minerals are often formed in water. A picture below shows an inverted channel in Miyamoto Crater. Inverted channels formed from accumulated sediments that were cemented by minerals. These channels eroded into the surface, and then the whole area was covered over with sediments. When the sediments were later eroded away, the place where the river channel existed remained because the hardened material that was deposited in the channel was resistant to erosion.[64] Iani Chaos, pictured below, was among the top 33 landing sites. Deposits of hematite and gypsum have been found there.[65] Those minerals are usually formed in connection with water.
The aim of the Mars Science Laboratory, later named Curiosity, is to search for signs of ancient life. It is hoped that a later mission could then return samples from sites that the Mars Science Laboratory identified as probably containing remains of life. To safely bring the craft down, a 12-mile-wide, smooth, flat circle must be found. Geologists hope to examine places where water once ponded.[66] They would like to examine sediment layers. In the end, it was decided to send the Mars science Laboratory, called "Curiosity," to Gale Crater in the Aeolis quadrangle."
Inverted relief
Some places on Mars show inverted relief. In these locations, a stream bed may be a raised feature, instead of a valley. The inverted former stream channels may be caused by the deposition of large rocks or due to cementation. In either case erosion would erode the surrounding land and leave the old channel as a raised ridge because the ridge will be more resistant to erosion. An image below, taken with HiRISE of Miyamoto Crater displays a ridge that is an old channel that has become inverted.[67]
Deltas
Researchers have found a number of examples of deltas that formed in Martian lakes. Deltas are major signs that Mars once had a lot of water. Deltas often require deep water over a long period of time to form. Also, the water level needs to be stable to keep sediment from washing away. Deltas have been found over a wide geographical range. Below, are pictures of a few.[68]
Craters
Jones Crater, as seen by CTX camera (on Mars Reconnaissance Orbiter). Regions on the floor containing layers, fans, and dunes are labeled.
Impact craters generally have a rim with ejecta around them; in contrast, volcanic craters usually do not have a rim or ejecta deposits. As craters get larger (greater than 10 km in diameter), they usually have a central peak.[69] The peak is caused by a rebound of the crater floor following the impact.
[70] Sometimes craters will display layers. Craters can show us what lies deep under the surface.
In December 2011, Opportunity Rover discovered a vein of gypsum sticking out of the soil along the rim of Endeavour crater. Tests confirmed that it contained calcium, sulfur, and water. The mineral gypsum is the best match for the data. It likely formed from mineral rich water moving through a crack in the rock. The vein, called "Homestake," is in Mars' Meridiani plain. It could have been produced in conditions more neutral than the harshly acidic conditions indicated by the other sulfate deposits; hence, this environment may have been more hospitable for a large variety of living organisms.[71]
Unnamed Channels
There is enormous evidence that water once flowed in river valleys on Mars.[72] [73] Images of curved channels have been seen in images from Mars spacecraft dating back to the early seventies with the Mariner 9 orbiter.[74] [75] [76] [77] A study published in June 2017, calculated that the volume of water needed to carve all the channels on Mars was even larger than the proposed ocean that the planet may have had. Water was probably recycled many times from the ocean to rainfall around Mars.[78][79]
Other landscapes in Margaritifer Sinus quadrangle
Light-toned deposit in Arsinoes Chaos, as seen by HiRISE under HiWish program. These kind of deposits often contain water bearing minerals like sulfates.
See also
References
- ↑ Davies, M.E.; Batson, R.M.; Wu, S.S.C. "Geodesy and Cartography" in Kieffer, H.H.; Jakosky, B.M.; Snyder, C.W.; Matthews, M.S., Eds. Mars. University of Arizona Press: Tucson, 1992.
- ↑ Blunck, J. 1982. Mars and its Satellites. Exposition Press. Smithtown, N.Y.
- ↑ Grotzinger, J. and R. Milliken (eds.) 2012. Sedimentary Geology of Mars. SEPM
- ↑ [http://linkinghub.elsevier.com/retrieve/pii/S0019103508002728 Fassett, C. and J. Head III. 2008. Valley network-fed, open-basin lakes on Mars: Distribution and implications for Noachian surface and subsurface hydrology. Icarus: 198. 39-56.
- ↑ [http://linkinghub.elsevier.com/retrieve/pii/S0019103500964650 Goldspiel, J. and S. Squyres. 2000. Groundwater sapping and valley formation on Mars. Icarus. 89: 176-192.
- ↑ Michael H. Carr|title=The surface of Mars|url=https://books.google.com/books?id=uLHlJ6sjohwC%7Caccessdate=21 March 2011|date=2006|publisher=Cambridge University Press|isbn=978-0-521-87201-0
- ↑ Cabrol, N. and E. Grin (eds.). 2010. Lakes on Mars. Elsevier. NY.
- ↑ Buhler, P. et al. 2011. Evidence for paleolakes in Erythracea Fossa, Mars: Implications for an ancient hydrological cycle. Icarus. 213: 104-115.
- ↑ Thomas, R., et al. 2017. EXTENSIVE EXPOSURE OF CLAY-BEARING NOACHIAN TERRAIN IN MARGARITIFER TERRA, MARS. Lunar and Planetary Science XLVIII. 2017. 1180.pdf
- ↑ Yen, A., et al. 2005. An integrated view of the chemistry and mineralogy of martian soils. Nature. 435.: 49-54.
- ↑ Bell, J (ed.) The Martian Surface. 2008. Cambridge University Press.
- ↑ Squyres, S. et al. 2004. The Opportunity Rover's Athena Science Investigation at Meridiani Planum, Mars. Science: 1698-1703.
- ↑ Soderblom, L., et al. 2004. Soils of Eagle Crater]and Meridiani Planum at the Opportunity Rover Landing Site. Science: 306. 1723-1726.
- ↑ Christensen, P., et al. Mineralogy at Meridiani Planum from the Mini-TES Experiment on the Opportunity Rover. Science: 306. 1733-1739.
- ↑ Goetz, W., et al. 2005. Indication of drier periods on Mars from the chemistry and mineralogy of atmospheric dust. Nature: 436.62-65.
- ↑ Goetz, W., et al. 2005. Indication of drier periods on mars from the chemistry and mineralogy of atmospheric dust. Nature: 436, 62-65.
- ↑ Bandfield, J., et al. 2003. Spectroscopic identification of carbonate minerals in the Martian dust. Science: 301, 1084-1087.
- ↑ Christensen, P., et al. 2004. Mineralogy at meridian Planum from the Mini-TES Experiment on the Opportunity Rover. Science: 306, 1733.
- ↑ Bell, J., et al. 2004. Pancam Multispectral Imaging Results from the Opportunity Rover at Meridiani Planum. Science: 306.1703-1708.
- ↑ Christensen, P., et al. 2004 Mineralogy at Meridiani Planum from the Mini-TES Experiment on the Opportunity Rover. Science: 306. 1733-1739.
- ↑ Squyres, S. et al. 2004. In Situ Evidence for an Ancient Aqueous Environment at Meridian Planum, Mars. Science: 306. 1709-1714.
- ↑ Hynek, B. 2004. Implications for hydrologic processes on Mars from extensive bedrock outcrops throughout Terra Meridiani. Nature: 431. 156-159.
- ↑ Dreibus,G. and H. Wanke. 1987. Volatiles on Earth and Mars: a comparison. Icarus. 71:225-240
- ↑ Squyers, S., et al. 2004. In Situ Evidence for an Ancient Aqueous Environment at Meridiani Planum Mars. Science: 306, 1709-1714.
- ↑ Rieder, R., et al. 2004. Chemistry of Rocks and Soils at Meridiani Planum from the Alpha Particle X-ray Spectrometer. Science. 306. 1746-1749
- ↑ http://www.nasa.gov/mission_pages/mer/news/mer20111207.html
- ↑ https://www.sciencedaily.com/releases/2012/01/120125093619.htm
- ↑ Klingelhofer, G. et al. 2004. Jarosite and Hematite at Meridiani Planum from Opportunity's Mossbauer Spectrometer. Science: 306. 1740-1745.
- ↑ Herkenhoff, K., et al. 2004. Evidence from Opportunity's Microscopic Imager for Water on Meridian Planum. Science: 306. 1727-1730
- ↑ Squyres, S. et al. 2004. In Situ Evidence for an Ancient Aqueous Environment at Meridian Planum, Mars. Science: 306. 1709-1714.
- ↑ Herkenhoff, K., et al. 2004. Evidence from Opportunity's Microscopic Imager for Water on Meridian Planum. Science: 306. 1727-1730
- ↑ Yen, A., et al. 2005. An integrated view of the chemistry and mineralogy of martian soils. Nature. 435.: 49-54.
- ↑ Squyres, S. et al. 2004. In Situ Evidence for an Ancient Aqueous Environment at Meridian Planum, Mars. Science: 306. 1709-1714.
- ↑ Squyres, S., et al. 2004. The Opportunity Rover’s Athena Science Investigation at Meridiani Planum, Mars. Science: 306, 1698-1703.
- ↑ Squyers, S., et al. 2004. The Opportunity Rover’s Athena Science Investigation at Meridiani Planum, Mars. Science: 306, 1698-1709.
- ↑ McSween, et al. 1994. Meteoritics:29, 757.
- ↑ Christensen, P., et al. 2004. Mineralogy at Meridani Planum from the Mini-TES Experiment on the Opportunity Rover. Science: 306, 1733-1739.
- ↑ Squyres, S., et al. 2009. Exploration of Victoria Crater by the Mars Rover Opportunity. Science: 1058-1061.
- ↑ Schroder,C., et al. 2008. J. Geophys. Res.: 113.
- ↑ Squyres, S. et al. 2004. In Situ Evidence for an Ancient Aqueous Environment at Meridian Planum, Mars. Science: 306. 1709-1714.
- ↑ Clark, B. et al. Chemistry and mineralogy of outcrops at Meridiani Planum. Earth Planet. Sci. Lett. 240: 73-94.
- ↑ Salvatore, M., M. Kraft1, C. Edwards, P. Christensen. 2015. Geologic History of margaritifer Basin, Mars: Evidence for a Prolonged Yet Episodic Hydrologic System. 46th Lunar and Planetary Science Conference (2015) 1463.pdf
- ↑ Squyres, S. et al. 2004. In Situ Evidence for an Ancient Aqueous Environment at Meridian Planum, Mars. Science: 306. 1709-1714.
- ↑ =Hugh H. Kieffer|title=Mars|url=https://books.google.com/books?id=NoDvAAAAMAAJ%7Caccessdate=7 March 2011|date=1992|publisher=University of Arizona Press|
- ↑ Raeburn, P. 1998. Uncovering the Secrets of the Red Planet Mars. National Geographic Society. Washington, D.C.
- ↑ Moore, P. et al. 1990. The Atlas of the Solar System. Mitchell Beazley Publishers, New York.
- ↑ https://www.britannica.com/science/sapping
- ↑ Buczkowski D. et al. 2010. LPS XLI Abstract #1458.
- ↑ Buczkowski D. et al. 2013. LPS XLIV Abstract #2331.
- ↑ Buczkowski, D., K. Seelos, C. Beck, and S. Murchie. 2015. POTENTIAL ALTERATION BY GROUNDWATER FLOW IN NW NOACHIS TERRA: GEOMORPHIC AND MINERALOGIC EVIDENCE IN NIRGAL AND HER DESHER VALLES. 46th Lunar and Planetary Science Conference 2271.pdf
- ↑ Sorrell, C. Rocks and Minerals. 1973. Golden Press. N.Y.
- ↑ (HiRISE image; Observation ID: PSP_0040261765)
- ↑ http://hirise.lpl.arizona.edu?PSP_008437_1750 |title=HiRISE | High Resolution Imaging Science Experiment |publisher=Hirise.lpl.arizona.edu?psp_008437_1750
- ↑ Grotzinger, J. and R. Milliken (eds.). 2012. Sedimentary Geology of Mars. SEPM.
- ↑ Weitz, C. et al. 2017. LIGHT-TONED MATERIALS OF MELAS CHASMA: EVIDENCE FOR THEIR FORMATION ON MARS. Lunar and Planetary Science XLVIII (2017) 2794.pdf
- ↑ Weitz C., et al. 2015. Icarus: 251: 291-314
- ↑ Weitz, C. 2016. Journal of Geophysical Research: Planets, 2016, 121(5): 805-835.
- ↑ Bishop, J., et al. 2013. What the ancient phyllosilicates at Mawrth Vallis can tell us about possible habitability on early Mars. Planetary and Space Science: 86, 130-149.
- ↑ Next Mars Rover's Landing Site Narrowed to 4 Choices. JR Minkel, 15 June 2010 (SPACE.com)
- ↑ Grant,J., et al. 2008. HiRISE imaging of impact megabreccia and sub-meter aqueous strata in Holden Crater, Mars. Geology. 36: 195-198.
- ↑ Cabrol, N. and E. Grin (eds.). 2010. Lakes on Mars. Elsevier. NY.
- ↑ NASA Narrows List of Next Mars Landing Sites. Irene Klotz, 21 November 2008. (Discovery News) https://web.archive.org/web/20090225074621/http://dsc.discovery.com/news/2008/11/21/mars-landing-sites-02.html
- ↑ Murchie, S. et al. 2009. A synthesis of Martian aqueous mineralogy after 1 Mars year of observations from the Mars Reconnaissance Orbiter. Journal of Geophysical Research: 114. Template:Doi
- ↑ HiRISE - High Resolution Imaging Science Experiment
- ↑ "Iani_Chaos">The Floods of Iani Chaos (Mars Odyssey THEMIS)
- ↑ http://themis.mars.asu.edu/feature/31
- ↑ Sinuous Ridges Near Aeolis Mensae (HiRISE image; Observation ID: PSP_002279_1735)
- ↑ Irwin III, R. et al. 2005. An intense terminal epoch of widespread fluvial activity on early Mars: 2. Increased runoff and paleolake development. Journal of Geophysical Research: 10. E12S15
- ↑ Stones, Wind, and Ice: A Guide to Martian Impact Craters. Compiled by Nadine G. Barlow, Virgil L. Sharpton
- ↑ Hugh H. Kieffer (1992). Mars. University of Arizona Press. ISBN 978-0-8165-1257-7.
- ↑ http://www.nasa.gov/home/hqnews/2011/dec/HQ_11-403_Mars_Rover_Gypsum.html
- ↑ Baker, V., et al. 2015. Fluvial geomorphology on Earth-like planetary surfaces: a review. Geomorphology. 245, 149–182.
- ↑ Carr, M. 1996. in Water on Mars. Oxford Univ. Press.
- ↑ Baker, V. 1982. The Channels of Mars. Univ. of Tex. Press, Austin, TX
- ↑ Baker, V., R. Strom, R., V. Gulick, J. Kargel, G. Komatsu, V. Kale. 1991. Ancient oceans, ice sheets and the hydrological cycle on Mars. Nature 352, 589–594.
- ↑ Carr, M. 1979. Formation of Martian flood features by release of water from confined aquifers. J. Geophys. Res. 84, 2995–300.
- ↑ Komar, P. 1979. Comparisons of the hydraulics of water flows in Martian outflow channels with flows of similar scale on Earth. Icarus 37, 156–181.
- ↑ http://spaceref.com/mars/how-much-water-was-needed-to-carve-valleys-on-mars.html
- ↑ Luo, W., et al. 2017. New Martian valley network volume estimate consistent with ancient ocean and warm and wet climate. Nature Communications 8. Article number: 15766 (2017). doi:10.1038/ncomms15766
Recommended reading
- Hugh H. Kieffer (1992). Mars. University of Arizona Press. ISBN 978-0-8165-1257-7.
- Squyers, S., et al. 2004. The Opportunity Rover’s Athena Science Investigation at Meridiani Planum, Mars. Science: 306, 1698-1709
- Opportunity Rover Press Kit
- Margaritifer_Sinus_quadrangle Wikipedia