Difference between revisions of "Oxia Palus quadrangle"
Line 162: | Line 162: | ||
== Outflow channels and chaos == | == Outflow channels and chaos == | ||
− | [[File:Hydaspischaossquares.jpg|right|Layered mesas in Hydaspis Chaos| | + | [[File:Hydaspischaossquares.jpg|right|Layered mesas in Hydaspis Chaos|500px|Layered mesas in Hydaspis Chaos]] |
Layered mesas in Hydaspis Chaos | Layered mesas in Hydaspis Chaos |
Revision as of 12:27, 18 January 2019
Article written by Jim Secosky. Jim is a retired science teacher who has used the Hubble Space Telescope, the Mars Global Surveyor, and HiRISE.
The Oxia Palus quadrangle is one of a series of 30 quadrangle maps of Mars. It is also called Mars Chart-11 (MC-11).[1] The quadrangle covers the region of 0° to 45° west longitude 315 E) and 0° to 30° north latitude on Mars.[2] This quadrangle covers parts of many regions: Chryse Planitia, Arabia Terra, Xanthe Terra, Margaritifer Terra, Meridiani Planum and Oxia Planum.
Contents
- 1 Major Features
- 2 Past and Future Landings
- 3 Possible Lakes
- 4 Layered sediments
- 5 Craters
- 6 Mojave crater
- 7 Firsoff Crater
- 8 Crommelin Crater
- 9 Danielson Crater
- 10 Vallis
- 11 Springs
- 12 Linear ridge networks
- 13 Outflow channels and chaos
- 14 Aram Chaos
- 15 Other landscapes in Oxia Palus quadrangle
- 16 References
- 17 See Also
- 18 Recommended reading
- 19 External links
Major Features
The major landscape features of the Oxia Palus quadrangle are Aram Chaos, Ares Vallis, Mawrth Vallis, Shalbatana Vallis, and several prominent craters (Danielson, Firsoff, and Crommelin). Some features found here are very common on Mars, but rare—if they exist at all—on the Earth. Chaos, and linear ridge networks are in this category. As on Earth, this region of Mars displays channels, layered terrain, and faults[3] This article aims to describe the location and major features of this quadrangle. When you finish reading it, you will know what it looks like from orbit and from the surface. Some HiRISE images may even show how it looks from the altitude of an airplane or a helicopter.
Layers in Dannielson Crater, as seen by HiRISE under HiWish program Black box shows how big a football field would be.
Past and Future Landings
There is much interesting geology in this region. [Mars Pathfinder] landed in the Oxia Palus quadrangle at 19.13 N 33.22 W (326.78 E), on July 4, 1997. Launched on a Delta II rocket on December 4, 1996. The Mars Pathfinder mission was used to demonstrate that NASA could send an exploratory mission to Mars for 1/15th of the cost of the Viking budget in the 1970's. The mission was developed at a cost of under $150 million in 3 years. Mars Pathfinder landed in Ares Vallis, Chryse Planitia, at 19 degrees 7'48" N and 33 degrees 13'12"W in the Oxia Palus quadrangle.[4] [5] One of the major mission objectives was to deliver a microrover safely to the surface in order to study the surface composition. The microrover onboard was known as Sojourner. Rocks and soil were probed with an Alpha Proton X-ray Spectrometer (APXS),[6] Sojourner was powered with solar panels and a non-rechargeable battery, which greatly limited night activities. When the batteries were depleted, it could not work at night.[7] Its lithium-thionyl chloride (LiSOCl2) batteries only generated 150 watt-hours.[8] The Mars Pathfinder Mission lasted from December 1996 to March 1998. Pathfinder carried out more than 15 chemical analyses of rocks and soil, besides collecting data on the weather. Information learned through the mission suggest that, in its past, Mars was warm and wet, with liquid water on its surface and a thicker atmosphere. Depletion of the spacecraft's battery along with a drop in the spacecraft's operating temperature was believed to be why we lost communications with Pathfinder in October 1997. The mission far surpassed its expected 30-day lifetime.[9]
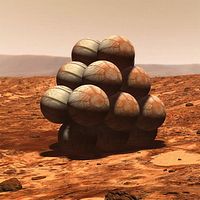
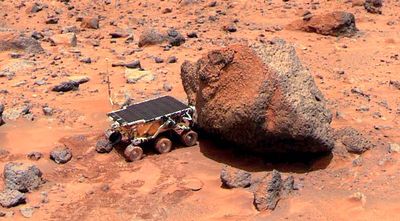
View from Mars Pathfinder
After several meetings of top scientists, it was decided that EXoMars 2020 will land in the Oxia Palus quadrangle at 18.14 N and 335.76 E. This site is of interest because of a long-duration aqueous system including a delta, possible biosignatures, and a variety of clays.[12] [13] [14] Another site, Mawrth Vallis, in this region was strongly considered as a landing site for NASA's Curiosity Mars Rover, which was in the end sent to Gale Crater.[15] [16] [17][18]
The Mawrth Vallis region is well studied with more than 40 papers published in peer-reviewed publications. Near the Mawrth channel is a 200 meter high plateau with many exposed layers. Spectral studies have detected clay minerals that present as a sequence of layers.[19] [20] [21] [22] [23] [24] [25] [26] [27]
Clay minerals detected in Mawrth were probably deposited in the Early to Middle Noachian period. Later weathering exposed a variety of minerals such as kaolin, alunite, and jarosite. Later, volcanic material covered the region. This volcanic material would have protected any possible organic materials from radiation.[28]
Possible Lakes
Research, published in January 2010, suggests that Mars had lakes, each around 20 km wide, along parts of the equator, in the Oxia Palus quadrangle. Although earlier research showed that Mars had a warm and wet early history that has long since dried up, these lakes existed in the Hesperian Epoch, a much earlier period. Using detailed images from NASA's Mars Reconnaissance Orbiter, the researchers speculate that there may have been increased volcanic activity, meteorite impacts, or shifts in Mars' orbit during this period to warm Mars' atmosphere enough to melt the abundant ice present in the ground. Volcanoes would have released gases that thickened the atmosphere for a temporary period, trapping more sunlight and making it warm enough for liquid water to exist. In this new study, channels were discovered that connected lake basins near Ares Vallis. When one lake filled up, its waters overflowed the banks and carved the channels to a lower area where another lake formed.[29] These lakes would be another place to look for evidence of present or past life. This quadrangle contains abundant evidence for past water in such forms as river valleys, lakes, springs, and chaos areas where water flowed out of the ground. A variety of clay minerals have been found in Oxia Palus. Clay is formed in water, and it is good for preserving microscopic evidence of ancient life.[30] Recently, scientists have found strong evidence for a lake located in the Oxia Palus quadrangle that received drainage from Shalbatana Vallis. The study, carried out with HiRISE images, indicates that water formed a 30-mile-long canyon that opened up into a valley, deposited sediment, and created a delta. This delta and others around the basin imply the existence of a large, long-lived lake. Of special interest is evidence that the lake formed after the warm, wet period was thought to have ended. So, lakes may have been around much longer than previously thought.[31] [32] In October 2015, Oxia Planum, a plain located near 18.275 N and 335.368 E was announced as the preferred landing site for the EXoMARS rover.[33] [34]
Layered sediments
Oxia Palus is an interesting area with many craters showing layered sediments.[35] Such sediments may have been deposited by water, wind, or volcanoes. The thickness of the layers is different in different craters. In Becquerel many layers are about 4 meters thick. In Crommelin crater the layers average 20 meters in thickness. At times, the top layer may be resistant to erosion and will form a feature called a Mensa , the Latin word for table.[36] One explanation put forth for the formation of at least some of the layers involves the changeable climate on Mars. The tilt of the Earth's axis changes by only a little more than 2 degrees. In contrast, Mars's tilt varies by tens of degrees. Today, the tilt (or obliquity) of Mars is low, so the poles are the coldest places on the planet, while the equator is the warmest. This causes gases in the atmosphere, like water and carbon dioxide, to migrate poleward, where they turn into ice. When the obliquity is higher, the poles receive more sunlight, and ices move away. Dry ice (carbon dioxide ice) turns into a gas thus raising atmospheric pressure. A thicker atmosphere may have more powerful winds capable of transporting and depositing more sand. Also, more water in the atmosphere, sand grains deposited on the surface may more readily stick and cement together to form layers. [37] A detailed discussion of layering with many Martian examples can be found in Sedimentary Geology of Mars.[38]
Craters
Image with significant craters and other features labeled
There are many large impact craters in this region. Several are named in honor of famous scientists. Besides Galileo Galilei and DaVinci, some of the names of people who discovered the atom and radiation have been used for crater names such as Marie Curie, Henri Becquerel, and Ernest Rutherford.[39]
Impact craters generally have rims with ejecta around them; in contrast volcanic craters usually do not have a rim or ejecta deposits. As impact craters get larger (greater than 10 km in diameter), they usually have a central peak.[40] The peak is caused by a rebound of the crater floor after the impact.[41] Sometimes craters display layers in their walls or in deposits on their floors. Since the collision that produces a crater is like a powerful explosion, rocks from deep underground are tossed onto the surface. Hence, craters can show what lies deep under the surface. Hence, the area around craters is a worthwhile place to gather rock samples.
Western side of Rutherford Crater, as seen by CTX camera (on Mars Reconnaissance Orbiter) Light-toned deposits are visible. Light-toned materials are associated with past water.
Mojave crater
The crater Mojave, in the Xanthe Terra region, has alluvial fans that look remarkably similar to landforms in the Mojave Desert in the American southwest. As on Earth, the largest rocks are near the mouths of the fans. Because channels start at the tops of ridges, it is believed they were formed by heavy downpours. Researchers have suggested that the rain may have been initiated by impacts.[42] Mojave is approximately 1.618 miles (2,604 meters) deep. Its depth relative to its diameter and its ray system indicate it is very young. Crater counts of its ejecta blanket give an age of about 3 million years. It is considered the most recent crater of its size on Mars, and has been identified as the probable source of the shergottite meteorites collected on Earth. [43]
Firsoff Crater
MOLA map showing Firsoff Crater and other nearby craters. Colors indicate elevations.
Crommelin Crater
Crommelin Crater showing layers arranged in ovals as seen by ctx
Danielson Crater
Wide view of layers in Danielson Crater Picture was taken by HiRISE under HiWish program.
Vallis
'Vallis (plural valles) is the Latin word for valley. It is used in planetary geology for the naming of river-like features on other planets. Vallis was used for old river valleys that were discovered on Mars, when probes were first sent to Mars. The Viking Orbiters caused a revolution in our conception of water on Mars; huge river valleys were found in many areas. As the years of study of Mars have gone on spacecraft cameras showed that floods of water broke through dams, carved deep valleys, eroded grooves into bedrock, and traveled thousands of kilometers.[44] [45] [46].[47] [48] [49] [50] [51] [52]
Ares Vallis, as seen by Viking. The channel is 25 km wide and about 1 km deep.
Springs
A study of images taken with the High Resolution Imaging Science Experiment (HiRISE) on the Mars Reconnaissance Orbiter strongly suggests that hot springs once existed in Vernal Crater, in the Oxia Palus quadrangle. These springs may have provided a long-time location for life. Furthermore, mineral deposits associated with these springs may have preserved traces of Martian life. In Vernal Crater on a dark part of the floor, there are two light-toned, elliptical structures that closely resemble hot springs on the Earth. They have inner and outer halos, with roughly circular depressions. A large number of hills are lined up close to the springs. These are thought to have formed by the movement of fluids along the boundaries of dipping beds. A picture below shows these springs. One of the depressions is visible. The discovery of opaline silica by the Mars Rovers, on the surface also suggests the presence of hot springs. Opaline silica is often deposited in hot springs.[53] Scientists proposed this area should be visited by the Mars Science Laboratory. [54]
Vernal Crater, as seen by CTX camera (on Mars Reconnaissance Orbiter).
Linear ridge networks
Linear ridge networks are found in various places on Mars in and around craters.[55] Ridges often appear as mostly straight segments that intersect in a lattice-like manner. They are hundreds of meters long, tens of meters high, and several meters wide. It is thought that impacts created fractures in the surface, these fractures later acted as channels for fluids. Fluids cemented the structures. With the passage of time, surrounding material was eroded away, thereby leaving hard ridges behind. Since the ridges occur in locations with clay, these formations could serve as a marker for clay which requires water for its formation.[56] [57] Water here could have supported past life in these locations. Clay may also preserve fossils or other traces of past life.
Outflow channels and chaos
Layered mesas in Hydaspis Chaos
Many large, ancient river valleys are found in this area; along with collapsed features, called Chaos. The Chaotic features may have collapsed when water came out of the surface. Martian rivers begin with a Chaos region. A chaotic region can be recognized by a jumble of mesas, buttes, and hills, chopped through with valleys which in places look almost patterned. Some parts of this chaotic area have not collapsed completely—they are still formed into large mesas, so they may still contain water ice.[58] Chaotic terrain occurs in numerous locations on Mars, and always gives the strong impression that something abruptly disturbed the ground. Chaos regions formed long ago. By counting craters (more craters in any given area means an older surface) and by studying the valleys' relations with other geological features, scientists have concluded the channels in Oxia Palus formed 2.0 to 3.8 billion years ago.[59]
One generally accepted understanding for the formation of large outflow channels is that they were formed by catastrophic floods of water released from giant groundwater reservoirs. Maybe, the water began to come out of the ground because of faulting or volcanic activity. Sometimes hot magma just travels under the surface. If that is the case, the ground will be heated, but there may be no evidence of lava on the surface. After water escapes, the surface collapses. Moving across the surface, the water would have simultaneously frozen and evaporated. Chunks of ice that would have quickly formed may have enhanced the erosive power of the flood. Furthermore, the water may have frozen over at the surface, but continuing to flow underneath, eroding the ground as it moved along. Rivers in cold climates on the Earth often become ice-covered, but continue to flow.
Such catastrophic floods have occurred on Earth. One commonly cited example is the Channeled Scabland of Washington State; it was formed by the breakout of water from the Pleistocene Lake Missoula, that resembles a Martian outflow channels.[60]
Water that originated from chaos regions may have ultimately flowed in a downstream direction to end up in a northern ocean that may have covered one third of the planet. [61] [62] [63] [64]
Chaos terrain on Mars is believed to be associated with the release of huge amounts of water. Many large outflow rivers begin with chaos regions. A chaotic region can be recognized by numerous mesas, buttes, and hills. Today, we generally believe that much of the water of early Mars formed a frozen ice layer (called a cryosphere). As the water froze to the bottom, the layer became thicker and pressurized a layer of water beneath in an aquifer. Perhaps after an asteroid impact cracked the cryosphere, catastrophic floods came out of chaos regions and carved great outflow channels that carried the water to a northern ocean. [65] [66] [67] [68]
Aram Chaos
Aram Chaos is an ancient impact crater about 170 miles (280 km) across, near the Martian equator, close to Ares Vallis. There is much evidence that water was here in the past. Aram lies in a region called Margaritifer Terra, where many water-carved channels show that floods poured out of the highlands onto the northern lowlands ages ago. The Thermal Emission Imaging System (THEMIS) on the Mars Odyssey orbiter found gray crystalline hematite on Aram’s floor. Hematite, an iron-oxide mineral, can precipitate when ground water circulates through iron-rich rocks. The floor of Aram contains huge blocks of collapsed, or chaotic, terrain that formed when water or ice was catastrophically removed. Elsewhere on Mars, the release of groundwater produced massive floods that eroded the large channels seen in Ares Vallis and other outflow valleys. In Aram Chaos, however, the released water stayed mostly within the crater's ramparts, eroding only a small, shallow outlet channel in the eastern wall. The presence of certain minerals, including hematite, sulfate minerals, and water-altered silicates, in Aram suggests that a lake probably once existed within the crater. Since the formation of hematite needs liquid water, which could not long exist without a thick atmosphere, the presence of hematite suggests that Mars must have had a much thicker atmosphere at some time in the past.[69]
Other landscapes in Oxia Palus quadrangle
The route taken on Mars from the movie “The Martian”
This map shows the route that ‘The Martian’, Mark Watney, travelled on Mars. Before he was able to leave his location at Chryse Planitia for the safety of Ares 4 in Schiaparelli Crater, he had to set up a radio transmitter that he obtained at the landing site of the first Mars rover, Soujourner, which arrived on the Red Planet in 1997 as part of the Mars Pathfinder mission. Sojourner was located a few hundred kilometers to the south of Chryse Planitia. His journey continued to the mouth of Mawrth Vallis, which he drove up and gained about 2000 meters in altitude. Following this, Watney drove an additional 2500 meters uphill through the rough Meridiani Planum, which is littered with craters, to the rim of the 450-kilometre diameter Schiaparelli Crater. On the northwestern rim of the crater, a landslide has created a natural ramp that Watney used to access the crater floor, some 700 meters below, where the Ares-4 rocket was 'parked'.[70] [71]
References
- ↑ Davies, M.E.; Batson, R.M.; Wu, S.S.C. "Geodesy and Cartography" in Kieffer, H.H.; Jakosky, B.M.; Snyder, C.W.; Matthews, M.S., Eds. Mars. University of Arizona Press: Tucson, 1992.
- ↑ https://planetarynames.wr.usgs.gov/
- ↑ https://en.wikipedia.org/wiki/Oxia_Palus_quadrangle
- ↑ https://en.wikipedia.org/wiki/Mars_Pathfinder
- ↑ https://www-k12.atmos.washington.edu/k12/mars/MPF_short_facts.html
- ↑ Cite journal |title=Determination of the chemical composition of Martian soil and rocks: The alpha proton X ray spectrometer |author1=R. Rieder |author2=H. Wänke |author3=T. Economou |author4=A. Turkevich |journal= Journal of Geophysical Research |date= 1997 |volume= 102 |pages=4027–4044 |doi=10.1029/96JE03918 |
- ↑ https://mars.jpl.nasa.gov/MPF/rover/faqs_sojourner.html
- ↑ cite web|url=http://mars.jpl.nasa.gov/MPF/rover/descrip.html%7Ctitle=Description of the Rover Sojourner|
- ↑ https://mars.nasa.gov/odyssey/files/odyssey/odysseyarrival1.pdf
- ↑ Golombek, M., et al. 1997. Overview of the Mars Pathfinder Mission and Assessment of Landing Site Predictions. Science: 278, 1743-1748.
- ↑ Golombek, M., et al. 1997. Overview of the Mars Pathfinder Mission and Assessment of Landing Site Predictions. Science: 278, 1743-1748.
- ↑ https://www.hou.usra.edu/meetings/lpsc2018/eposter/2177.pdf
- ↑ Quantin C. et al. (2014) ExoMars LSSW#1
- ↑ http://exploration.esa.int/mars/
- ↑ https://mars.nasa.gov/msl/mission/timeline/prelaunch/landingsiteselection/mawrthvallis2/
- ↑ https://hirise.lpl.arizona.edu/PSP_006610_2035
- ↑ https://mars.jpl.nasa.gov/resources/3564/mawrth-vallis-a-finalist-not-selected-as-a-landing-site-for-curiosity
- ↑ http://www.geo.brown.edu/geocourses/geo292/papers/Michalskietal2010.pdf
- ↑ Poulet; et al. (2005). "Phyllosilicates on Mars and implications for early martian climate". Nature. 438: 623–627.
- ↑ Loizeau et al. 2007. JGR 112, E08S08
- ↑ Bishop; et al. (2008). "Phyllosilicate Diversity and Past Aqueous Activity Revealed at Mawrth Vallis, Mars". Science. 321: 830.
- ↑ Loizeau; et al. (2010). "Stratigraphy in the Mawrth Vallis region through OMEGA, HRSC color imagery and DTM". Icarus. 205: 396–418.
- ↑ Farrand; et al. (2009). "Discovery of jarosite within the Mawrth Vallis region of Mars: Implications for the geologic history of the region". Icarus. 204: 478–488.
- ↑ Wray; et al. (2010). "Identification of the Ca-sulfate bassanite in Mawrth Vallis, Mars". Icarus. 209: 416–421.
- ↑ Bishop; et al. (2013). "What the Ancient Phyllosilicates at Mawrth Vallis can tell us about Possible Habitability on Early Mars". PSS. 86: 130–149.
- ↑ Michalski; et al. (2013). "Multiple working hypotheses for the formation of compositional stratigraphy on Mars: Insights from the Mawrth Vallis region". Icarus. 226: 816–840.
- ↑ Michalski; et al. (2010). "The Mawrth Vallis Region of Mars: A Potential Landing Site for the Mars Science Laboratory (MSL) Mission". Astrobio. 10: 687–703.
- ↑ Gross, C. et al. 2016. MAWRTH VALLIS – PROPOSED LANDING SITE FOR EXOMARS 2018/2020. 47th Lunar and Planetary Science Conference (2016) 1421.pdf
- ↑ Sanjeev Gupta, Nicholas Warner, Jung-Rack Kim, Shih-Yuan Lin, Jan Muller. 2010. Hesperian equatorial thermokarst lakes in Ares Vallis as evidence for transient warm conditions on Mars. Geology: 38. 71-74.
- ↑ http://themis.asu.edu/features/marwrthvillis
- ↑ http://www.colorado.edu/news/r/7e9c22ec0cd6dabc007bb14ed2e29f16.html
- ↑ https://www.space.com/6858-evidence-ancient-mars-lake.html
- ↑ https://hirise.lpl.arizona.edu/ESP_039154_1985
- ↑ https://www.universetoday.com/123018/scientists-want-exomars-rover-to-land-at-oxia-planum/
- ↑ Grotzinger, J. and R. Milliken (eds.) 2012. Sedimentary Geology of Mars. SEPM
- ↑ http://themis.asu.edu/zoom-20050314a.html
- ↑ http://www.spaceref.com:80/news/viewpr.html.pid=27101
- ↑ Grotzinger, J. and R. Milliken (eds.). 2012. Sedimentary Geology of Mars. SEPM.
- ↑ U.S. department of the Interior U.S. Geological Survey, Topographic Map of the Eastern Region of Mars M 15M 0/270 2AT, 1991
- ↑ http://www.lpi.usra.edu/publications/slidesets/stones/
- ↑ Kieffer, H., et al. (eds) 1992. Mars. The University of Arizona Press. Tucson
- ↑ http://hirise.lpl.arizona.edu/PSP_001415_1875
- ↑ "The Source Crater of Martian Shergottite Meteorites" (2014-03-06). Science 343: 1343–1346. doi: . PMID 24603150. Bibcode: 2014Sci...343.1343W.
- ↑ Kieffer, H., et al. (eds) 1992. Mars. The University of Arizona Press. Tucson
- ↑ Raeburn, P. 1998. Uncovering the Secrets of the Red Planet Mars. National Geographic Society. Washington D.C.
- ↑ Moore, P. et al. 1990. The Atlas of the Solar System. Mitchell Beazley Publishers NY, NY.
- ↑ Baker, V., et al. 2015. Fluvial geomorphology on Earth-like planetary surfaces: a review. Geomorphology. 245, 149–182.
- ↑ Carr, M. 1996. in Water on Mars. Oxford Univ. Press.
- ↑ Baker, V. 1982. The Channels of Mars. Univ. of Tex. Press, Austin, TX
- ↑ Baker, V., R. Strom, R., V. Gulick, J. Kargel, G. Komatsu, V. Kale. 1991. Ancient oceans, ice sheets and the hydrological cycle on Mars. Nature 352, 589–594.
- ↑ Carr, M. 1979. Formation of Martian flood features by release of water from confined aquifers. J. Geophys. Res. 84, 2995–300.
- ↑ Komar, P. 1979. Comparisons of the hydraulics of water flows in Martian outflow channels with flows of similar scale on Earth. Icarus 37, 156–181.
- ↑ Allen, C.; Oehler, D. (2008). "A Case for Ancient Springs in Arabia Terra, Mars". Astrobiology. 8: 1093–1112.
- ↑ http://hirise.lpl.arizona.edu/PSO_002812_1855
- ↑ Head, J., J. Mustard. 2006. Breccia dikes and crater-related faults in impact craters on Mars: Erosion and exposure on the floor of a crater 75 km in diameter at the dichotomy boundary, Meteorit. Planet Science: 41, 1675-1690.
- ↑ Mangold; et al. (2007). "Mineralogy of the Nili Fossae region with OMEGA/Mars Express data: 2. Aqueous alteration of the crust". J. Geophys. Res. 112. B
- ↑ Mustard et al., 2007. Mineralogy of the Nili Fossae region with OMEGA/Mars Express data: 1. Ancient impact melt in the Isidis Basin and implications for the transition from the Noachian to Hesperian, J. Geophys. Res., 112.
- ↑ http://themis.asu.edu/features/aramchaos
- ↑ http://themis.asu.edu/features/hydraotes
- ↑ http://www.msss.com/http/ps/channels/channels.html
- ↑ Clifford, S. M.; Parker, T. J. (2001). "The Evolution of the Martian Hydrosphere: Implications for the Fate of a Primordial Ocean and the Current State of the Northern Plains". Icarus. 154 (1): 40–79.
- ↑ Gulick, V., D. Tyler, C. McKay, and R. Haberle. 1997. Episodic ocean‐induced CO2 greenhouse on Mars: Implications for fluvial valley formation. Icarus: 130, 68–86.
- ↑ Andrews-Hanna, J., R. Phillips. 2007. Hydrological modeling of outflow channels and chaos regions on Mars. Journal of Geophysical Research: Planets Volume 112, Issue E8
- ↑ Clifford, S. 1993. A model for the hydrologic and climatic behavior of water on Mars. JGR, 98, 10973L
- ↑ Clifford, S. M.; Parker, T. J. (2001). "The Evolution of the Martian Hydrosphere: Implications for the Fate of a Primordial Ocean and the Current State of the Northern Plains". Icarus. 154 (1): 40–79. Bibcode:2001Icar..154...40C. doi:10.1006/icar.2001.6671
- ↑ Gulick, V., D. Tyler, C. McKay, and R. Haberle. 1997. Episodic ocean‐induced CO2 greenhouse on Mars: Implications for fluvial valley formation. Icarus: 130, 68–86.
- ↑ Andrews-Hanna, J., R. Phillips. 2007. Hydrological modeling of outflow channels and chaos regions on Mars. Journal of Geophysical Research: Planets Volume 112, Issue E8
- ↑ Clifford, S. 1993. A model for the hydrologic and climatic behavior of water on Mars. JGR, 98, 10973L
- ↑ http://themis.asu.edu/discoveries-aramchaos
- ↑ https://commons.wikimedia.org/wiki/File:The_route_of_%27The_Martian%27_-_from_Chryse_Planitia_over_Arabia_Terra_in_the_Martian_highlands_to_Ares_4.jpg
- ↑ https://en.wikipedia.org/wiki/The_Martian_(film)
See Also
- Geography of Mars
- High Resolution Imaging Science Experiment (HiRISE)
- Layers on Mars
- Oceans on Mars
- Rivers on Mars
Recommended reading
- Grotzinger, J., R. Milliken (eds.). 2012. Sedimentary Geology of Mars. Tulsa: Society for Sedimentary Geology.
- Kieffer, H., et al. (eds) 1992. Mars. The University of Arizona Press. Tucson
External links
- https://www.youtube.com/watch?v=zUaalbRC7KA Mars Pathfinder mission - LIVE coverage - 1997 - part 1
- https://www.youtube.com/watch?v=9HGRReKUzfU NASA's Mars Pathfinder & Sojourner Rover (360 View) (2 minutes)
- https://www.youtube.com/watch?v=HrPfcv8tB9A Mars Pathfinder - Video Learning - WizScience.com (2 minutes)
- https://www.youtube.com/watch?v=0Zy-n9x09oQ Oxia Planum & Mawrth Vallis - ExoMars 2020 rover landing site candidates
- https://www.youtube.com/watch?v=UqPsu230YKQ Oxia Palus quadrangle - Video Learning - WizScience.com
- https://www.youtube.com/watch?v=ZvhHiY4oU44 Danielson Crater, Mars