Difference between revisions of "Terraforming"
(Added section on regular greenhouse gases and a bit more detail on other methods of warming.) |
(→Partial terraforming: Even adding 10 mbar would allow liquid water.) |
||
(24 intermediate revisions by 2 users not shown) | |||
Line 18: | Line 18: | ||
Regular [[greenhouse gases]] such as water or CH4 (methane) will build up as Mars warms and the air pressure increases. Methane is produced by life, so even simple life (anorexic bacteria) will help terraform the planet, making a positive feedback loop. | Regular [[greenhouse gases]] such as water or CH4 (methane) will build up as Mars warms and the air pressure increases. Methane is produced by life, so even simple life (anorexic bacteria) will help terraform the planet, making a positive feedback loop. | ||
+ | |||
+ | Nano particles who's length is about 1/2 the length of infrared light would have a strong warming potential. This study <ref>https://www.science.org/doi/10.1126/sciadv.adn4650|Feasiblity of Keeping Mars Warm With Nano-particles</ref>, suggests that conducting nano rods, 9 micrometers long could warm mars more than a thousand times more effectively than greenhouse gases. If such tiny rods were made out of aluminium or iron they would be lifted like dust into the air, and remain effective for about 10 years. A significant advantage of this is that aluminum & iron are common on Mars, whereas super greenhouse gases need fluorine which is rare. See [[Greenhouse nano-particles]] for more information. | ||
Using nuclear bombs to vaporize the southern carbon dioxide (CO2) ice cap is now thought to be ineffective. (The CO2 would simply freeze out again.) NASA researcher, Chris McKay, has pointed out that all the nuclear bombs on Earth only equal the energy of 1/2 an hour of Martian sunlight. Global warming is caused by retaining more of the Sun's heat, not with nuclear bombs. | Using nuclear bombs to vaporize the southern carbon dioxide (CO2) ice cap is now thought to be ineffective. (The CO2 would simply freeze out again.) NASA researcher, Chris McKay, has pointed out that all the nuclear bombs on Earth only equal the energy of 1/2 an hour of Martian sunlight. Global warming is caused by retaining more of the Sun's heat, not with nuclear bombs. | ||
Line 24: | Line 26: | ||
− | A life supporting atmosphere needs to contain a "buffer gas", such as nitrogen. Mars is currently lacking in nitrogen, but nitrogen could be sourced from Venus, Saturn's moon Titan, or from comets. (Note that it is likely that Mars has nitrogen in its soil, see [[Atmospheric loss]].) Nitrogen is transparent to heat radiation, so it is not a greenhouse gas. | + | A life supporting atmosphere needs to contain a "buffer gas", such as nitrogen. Mars is currently lacking in nitrogen, but nitrogen could be sourced from Venus, Saturn's moon Titan, or from comets. (Note that it is likely that Mars has nitrogen in its soil, see [[Atmospheric loss]].) Nitrogen is transparent to heat radiation, so it is not a greenhouse gas. Mars has about 3% nitrogen in its atmosphere, so the nitrogen partial pressure is about 0.00018 bar. |
Curiosity rover detected nitrates in the soil of Mars. If widespread, this is very good news for terraformers, as the nitrogen is biologically available and if moved into the atmosphere, would give Mars approximately 0.3 Bar of Nitrogen partial pressure. <ref>https://agupubs.onlinelibrary.wiley.com/doi/full/10.1002/2016GL072199 - Measurements of Nitrates and Perchlorates in Martian soil</ref> | Curiosity rover detected nitrates in the soil of Mars. If widespread, this is very good news for terraformers, as the nitrogen is biologically available and if moved into the atmosphere, would give Mars approximately 0.3 Bar of Nitrogen partial pressure. <ref>https://agupubs.onlinelibrary.wiley.com/doi/full/10.1002/2016GL072199 - Measurements of Nitrates and Perchlorates in Martian soil</ref> | ||
==Pioneer Organisms== | ==Pioneer Organisms== | ||
− | The first creatures to live on the natural Mars landscape would be bacteria. Cyanobacteria (aka blue-green algae), live in water and would produce oxygen. They can live under ice if the ice cover is not too thick. | + | The first creatures to live on the natural Mars landscape would be bacteria. (See [[Bacteria Colonists]].) Cyanobacteria (aka blue-green algae), live in water and would produce oxygen. They can live under ice if the ice cover is not too thick. |
+ | |||
+ | A desert moss known as Syntrichia Caninervis, is drought and cold resistant. This extremophile was stored in liquid nitrogen (-196C) for one month. After being returned to room temperature, all samples recovered within one month. Placed in an arid environment (where 98% of the water was sucked out), the moss turned black and looked dead. But when given water, it recovered, looking green within seconds, and the deeper cells had rehydrated within minutes. It can photosynthesize even while covered by snow. Exposed to gamma radiation, the half lethal dose is estimated to be 5,000 Sv. This is a MASSIVE radiation dose, 4 Sievert are likely a lethal dose for humans, tho many plants can survive 500 Sv. (However, Mars is not exposed to high gamma rays doses, more useful information would be how well this species survives long exposure to UV radiation.) When placed in a Mars Jar (which simulates Martian conditions including UV radiation) for 1 week, it survived. However, it didn't grow, and only revived after being returned to an Earth environment.<ref>https://www.cell.com/the-innovation/fulltext/S2666-6758(24)00095-X</ref> | ||
Certain organisms, such as [[archaea]], [[lichen]], and [[tardigrades]] have been proven capable of surviving for some time in extreme environments, such as the vacuum of space. They could gain a foothold on the Martian surface after minimal terraforming. The byproducts of their metabolism would contribute to the terraforming efforts. | Certain organisms, such as [[archaea]], [[lichen]], and [[tardigrades]] have been proven capable of surviving for some time in extreme environments, such as the vacuum of space. They could gain a foothold on the Martian surface after minimal terraforming. The byproducts of their metabolism would contribute to the terraforming efforts. | ||
==Calculations== | ==Calculations== | ||
− | To increase Martian air pressure to 25 kPa, 2500 kg per m2 of atmosphere would be required. This is equivalent to a layer of about 1,5m of solid CO2. | + | *To increase Martian air pressure to 25 kPa, 2500 kg per m2 of atmosphere would be required. This is equivalent to a layer of about 1,5m of solid CO2. However, since this is not available on Mars, the existing CO2 would need to be supplemented by an inert gas, such as nitrogen, that might be sourced from Venus, comets or the outer solar system such as Saturn's moon titan. To round out the use of existing CO2, at least 2-3e17 kg of nitrogen would need to be imported. If the importation deltaV was 10 km/s, then the entire amount solar energy falling on Mars for about 20 years would be required to move the nitrogen to Mars. |
+ | **If importing from Venus, most of the energy would be spend moving the nitrogen up to Venus low orbit (9.2 km/s), for a total deltaV of about 15 km/s. | ||
+ | **If imported from Titan, the total deltaV would be about 12 km/s. | ||
+ | **If imported from Ceres, the total deltaV might be 8 km/s (7.5 km/s for asteroids with trivial escape velocities). Note that the outer edge of the asteroid belt (nearer to Jupiter) is beyond the ice line, so they will have more water and more elements that dissolve in water. | ||
+ | **Asteroids in Kuiper belt (richer in volatiles) are likely to have nitrogen in the form of [[Ammonia]] ice. However, the delta vee to move elements this deep into the sun's gravity well is huge (~23 km/s). However, a low cost method such as magnetic sails might work, given plenty of time. | ||
+ | |||
+ | |||
+ | *The water available on Mars in frozen form is already sufficient for a significative ocean. | ||
− | The | + | *Drawing down the CO2 partial pressure is likely to be done by biological carbon sequestration. Carbon sequestration in a biosphere might store between 100 to 300 tons of carbon per hectare, or 10-30 kg/m2 (ref). Carbon sequestration would consume CO2 and free oxygen, increasing its concentration in the atmosphere. The carbon in the Martian atmosphere masses about 6.5e15 kg. This would be enough to supply 2 to 6e14 m2 of land with the required sequestrated carbon. As Mars' surface is only 1.44e14m2, the biosphere could absorb most, but not all, of the carbon. Additional CO2 would be stored in a Martian ocean, or as carbonates. Therefore excess CO2 is probably not a problem, the problem is adding the required buffer gas, most likely nitrogen. Some CO2 would need to remain in the atmosphere to run the CO2 cycle. |
+ | |||
+ | Note that covering the entire planet with soil, and then adding carbon to it is very slow. Fogg has suggested that growing trees, and moving the lumber outside the biosphere (say by burying it deep, or placing it above the atmosphere in the Olympus Mons caldera) would be a much faster way of drawing down CO2 levels, taking only thousands of years. | ||
==Long term prospects== | ==Long term prospects== | ||
− | The ultimate results of terraforming are disputed. Terraforming may have only a temporary effect, even if the effect lasts for some hundred or thousand years. Eventually, the [[solar wind]] may carry away most of the new atmosphere due to the insufficient [[magnetosphere|magnetic field]]s of Mars, though this would take millions of years. (See [[Atmospheric loss]] for more details.) | + | The ultimate results of terraforming are disputed. Terraforming may have only a temporary effect, even if the effect lasts for some hundred or thousand years. Eventually, the [[solar wind]] may carry away most of the new atmosphere due to the insufficient [[magnetosphere|magnetic field]]s of Mars, though this would take hundreds of millions of years. (See [[Atmospheric loss]] for more details.) |
− | It has been suggested that the cost of terraforming a planet would be prohibitive. However, to a growing population on the surface of that planet it would most likely be considered a normal colonial function to ensure that daily colonial | + | It has been suggested that the cost of terraforming a planet would be prohibitive. However, to a growing population on the surface of that planet it would most likely be considered a normal colonial function to ensure that daily colonial endeavours have a positive effect on the atmosphere. Also note that industry will naturally produce greenhouse and super greenhouse gases for 'free', so some terraforming is likely to happen just from having a human presence on Mars. |
− | Artificial magnetic fields might also be created around Mars to reduce atmospheric losses to space. And the solar system contains sufficient resources to replenish Martian atmosphere indefinitely, but at a significant energy cost. Building space habitats might be a more practical long term objective for human occupation of space. | + | Artificial magnetic fields might also be created around Mars to reduce atmospheric losses to space. And the solar system contains sufficient resources to replenish Martian atmosphere indefinitely, but at a significant energy cost. Building space habitats might be a more practical long term objective for human occupation of space. Although space habitats may leak air faster than atmospheric loss from solar wind sputtering. |
==Partial terraforming== | ==Partial terraforming== | ||
Line 77: | Line 90: | ||
|} | |} | ||
− | While full terraforming to make Mars atmosphere suitable for breathable condition for humans | + | While full terraforming to make Mars atmosphere suitable for breathable condition for humans will take hundreds perhaps thousands of generations, transformations to the atmosphere suitable for plants could be faster. Current requirements for plants to grow on Mars are based on atmospheric pressure. Mars polar caps have enough CO<sub>2</sub> to provide 100 mbar (10 kPa) additional atmospheric pressure to the existing 6 millibars. This would probably be enough to create sustainable growth condition for plants. |
− | + | Note that even adding 10 mbar of pressure would make liquid water stable over much of the planet (if locally it was warm enough). This would allow bacteria and perhaps lichen to grow at times. | |
− | + | The first job will be to add bacteria. Many types can live at Mars' current pressures, but they need liquid water, and thus the temperature must be increased. A key goal is to warm the planet by about 20 degrees C. This will cause the poles to evaporate, releasing massive amounts of CO2, and a good deal of water. The increased pressure will result in liquid water appearing for short times (typically it will freeze at night). But even transient water is enough for bacteria to start spreading. See [[Bacteria Colonists]] for more information. | |
− | We would want to increase CO2 partial pressure to warm the planet, but would have to later draw down this CO2 level if we wish to make the | + | To add enough pressure to make using pressure suit unnecessary for humans, atmospheric pressure needs to rise to at least 100 mbar (10 kPa) or ~10% of Earth atmospheric pressure. |
+ | |||
+ | To make it so a breathing mask is not required, the pressure needs to be at least 250 mbar (25% Earth's pressure), AND the oxygen partial pressure must be increased, AND the carbon dioxide partial pressure must be reduced. Drawing down the CO2 partial pressure is likely to be done by biological carbon sequestration. Carbon sequestration in a biosphere might store between 100 to 300 tons of carbon per hectare, or 10-30 kg/m2 (ref). Carbon sequestration would consume CO2 and free oxygen, increasing its concentration in the atmosphere. | ||
+ | |||
+ | To get to a breathable atmosphere, a combination of oxygen, and various other gases must be generated. This might be composed of 118 mbar of nitrogen, 1 mbar of argon and neon, 1 mbar of water vapour and 130 mbar of oxygen (minimal requirement oxygen pressure). Note that the CO2 must be at trace levels for animals to breath. CO2 of 0.05% is the maximum we would want for a breathable atmosphere. (Argon and neon are not needed for life, but can act as a buffer gas.) | ||
+ | |||
+ | We would want to increase CO2 partial pressure to warm the planet, but would have to later draw down this CO2 level if we wish to make the planet habitable for animals. | ||
+ | |||
+ | Note that a partially terraformed world is still of use to humans. If we were to increase the pressure of the planet to 80 millibars, that is not enough for people to go on the surface with oxygen masks; they would need a pressure suit over most of the planet. But the LOWEST areas of the planet (e.g. the northern plains and Hellas Basin), would have high enough pressure to allow colonists to dispense with space suits and move about on the surface with simple gas masks. Further, the radiation from space, and solar UV would be reduced. (Not to Earth levels of safety, but better than current conditions.) Terraforming does not have to be 100% complete, to give safety and economic benefits to the habitants. | ||
[[Paraterraforming]] is a subset of terraforming where terraforming takes place inside huge structures which cover some fraction of the planet's surface. | [[Paraterraforming]] is a subset of terraforming where terraforming takes place inside huge structures which cover some fraction of the planet's surface. | ||
+ | |||
+ | ==Ethics of Terraforming== | ||
+ | This seems very straightforward to me (Rick). The universe is FILLED with sterile rocks. Life is rare and precious. Adding another world where life can live for millions or billions of years is a noble act of the highest order. Perhaps the 'purpose' of intelligent life is to spread life in all its forms across space. | ||
+ | |||
==Books on Terraforming== | ==Books on Terraforming== | ||
Line 97: | Line 122: | ||
<references /> | <references /> | ||
+ | x- https://cca-reports.ca/wp-content/uploads/2021/03/Carbon-Sinks_EN_CH-3_Forests.pdf | ||
[[category:Terraforming]] | [[category:Terraforming]] |
Latest revision as of 18:33, 25 November 2024
Terraforming, or Earth-shaping, is a theoretical process of modifying a planet's atmosphere to make it habitable for humans. In the case of Mars, terraforming would require first warming the planet, then artificial thickening of the atmosphere so pressure suits are not needed, ice melting to increase the H2O content of the atmosphere (creating water clouds), adding nitrogen as a buffer gas, and greatly increasing the O2 density to ultimately make the atmosphere breathable.
Nicole Willett has written her own article on Terraforming Mars.
Contents
Mars and the "Triple Point" of water
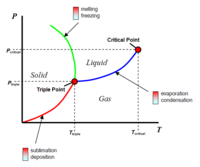
Presently, ice on Mars usually sublimes (rather than melts) as the atmospheric pressure is so low, ice bypasses the liquid phase when heated. Sublimation occurs allowing ice to turn directly into gas (steam). One of the main challenges for future terraforming efforts would be to increase the atmospheric pressure significantly so water can exist as a liquid on the surface of Mars. The atmospheric pressure and ambient temperature will therefore need to be greater than the triple point of water (thereby existing as ice, liquid and gas). This is just above 0C and 600 Pa. Mars atmospheric pressure is already above 600 Pa. However, close to the triple point, water takes very little energy to turn into a gas, so higher pressures would be required in practice.
Methods
Using giant mirrors to reflect light on to Mars would help warm Mars by increasing its insolation. Statites (stationary satellites held up by light pressure) have been suggested to beam light to the north and south poles of Mars. Others have suggested mirrors in sun synchronous orbits to beam light onto the terminator (which would brighten both dawn and dusk). An advantage of space mirrors is that they could be adjusted to direct light and heat to where it is most needed. (e.g. to the northern hemisphere in norther winter, and the Southern Hemisphere in southern winter.)
Mars could be warmed up using Super Greenhouse Gases such as perfluorocarbons, which are stable in the atmosphere for long periods of time. Since this method makes the heat which reaches Mars remain longer, it is very useful, and makes other strategies work better. (For example, space mirrors heating effect would be greater if the atmosphere held that heat longer.) It is likely that any plan to terraform Mars will use some Super Greenhouse Gases.
Other super greenhouse gasses include sulfur hexafluoride and 1,1,1-Trichloro ethane. These are very stable and highly effective greenhouse gasses. Use of such gasses to warm the atmosphere would allow the Carbon dioxide frozen into the polar caps and some of the water to evaporate adding to the mass of the atmosphere. If 4 hundredths of a microbar of manufactured greenhouse gas is needed to warm Mars to the point of runaway greenhouse effect, then a mass of manufactured greenhouse gasses equal to about 5.73 times the cargo capacity of the Edmund Fitzgerald (26,000 tonnes) every week for twenty years (about 150 million tonnes) would be required for the project. (Other estimates suggest a somewhat higher amount would be required to make up for the break up of these gases by ultraviolet light. See the Super Greenhouse Gas page for more discussion.)
Regular greenhouse gases such as water or CH4 (methane) will build up as Mars warms and the air pressure increases. Methane is produced by life, so even simple life (anorexic bacteria) will help terraform the planet, making a positive feedback loop.
Nano particles who's length is about 1/2 the length of infrared light would have a strong warming potential. This study [1], suggests that conducting nano rods, 9 micrometers long could warm mars more than a thousand times more effectively than greenhouse gases. If such tiny rods were made out of aluminium or iron they would be lifted like dust into the air, and remain effective for about 10 years. A significant advantage of this is that aluminum & iron are common on Mars, whereas super greenhouse gases need fluorine which is rare. See Greenhouse nano-particles for more information.
Using nuclear bombs to vaporize the southern carbon dioxide (CO2) ice cap is now thought to be ineffective. (The CO2 would simply freeze out again.) NASA researcher, Chris McKay, has pointed out that all the nuclear bombs on Earth only equal the energy of 1/2 an hour of Martian sunlight. Global warming is caused by retaining more of the Sun's heat, not with nuclear bombs.
However, using bombs to free nitrogen from nitrate deposits might be a useful technique. Martin J. Fogg in his Terraforming text book suggests using 'clean pipe' fusion bombs triggered with shaped explosives (rather than fission bomb triggers), to free nitrogen and ice from deep deposits, without the fallout of the fission bomb triggers.
A life supporting atmosphere needs to contain a "buffer gas", such as nitrogen. Mars is currently lacking in nitrogen, but nitrogen could be sourced from Venus, Saturn's moon Titan, or from comets. (Note that it is likely that Mars has nitrogen in its soil, see Atmospheric loss.) Nitrogen is transparent to heat radiation, so it is not a greenhouse gas. Mars has about 3% nitrogen in its atmosphere, so the nitrogen partial pressure is about 0.00018 bar.
Curiosity rover detected nitrates in the soil of Mars. If widespread, this is very good news for terraformers, as the nitrogen is biologically available and if moved into the atmosphere, would give Mars approximately 0.3 Bar of Nitrogen partial pressure. [2]
Pioneer Organisms
The first creatures to live on the natural Mars landscape would be bacteria. (See Bacteria Colonists.) Cyanobacteria (aka blue-green algae), live in water and would produce oxygen. They can live under ice if the ice cover is not too thick.
A desert moss known as Syntrichia Caninervis, is drought and cold resistant. This extremophile was stored in liquid nitrogen (-196C) for one month. After being returned to room temperature, all samples recovered within one month. Placed in an arid environment (where 98% of the water was sucked out), the moss turned black and looked dead. But when given water, it recovered, looking green within seconds, and the deeper cells had rehydrated within minutes. It can photosynthesize even while covered by snow. Exposed to gamma radiation, the half lethal dose is estimated to be 5,000 Sv. This is a MASSIVE radiation dose, 4 Sievert are likely a lethal dose for humans, tho many plants can survive 500 Sv. (However, Mars is not exposed to high gamma rays doses, more useful information would be how well this species survives long exposure to UV radiation.) When placed in a Mars Jar (which simulates Martian conditions including UV radiation) for 1 week, it survived. However, it didn't grow, and only revived after being returned to an Earth environment.[3]
Certain organisms, such as archaea, lichen, and tardigrades have been proven capable of surviving for some time in extreme environments, such as the vacuum of space. They could gain a foothold on the Martian surface after minimal terraforming. The byproducts of their metabolism would contribute to the terraforming efforts.
Calculations
- To increase Martian air pressure to 25 kPa, 2500 kg per m2 of atmosphere would be required. This is equivalent to a layer of about 1,5m of solid CO2. However, since this is not available on Mars, the existing CO2 would need to be supplemented by an inert gas, such as nitrogen, that might be sourced from Venus, comets or the outer solar system such as Saturn's moon titan. To round out the use of existing CO2, at least 2-3e17 kg of nitrogen would need to be imported. If the importation deltaV was 10 km/s, then the entire amount solar energy falling on Mars for about 20 years would be required to move the nitrogen to Mars.
- If importing from Venus, most of the energy would be spend moving the nitrogen up to Venus low orbit (9.2 km/s), for a total deltaV of about 15 km/s.
- If imported from Titan, the total deltaV would be about 12 km/s.
- If imported from Ceres, the total deltaV might be 8 km/s (7.5 km/s for asteroids with trivial escape velocities). Note that the outer edge of the asteroid belt (nearer to Jupiter) is beyond the ice line, so they will have more water and more elements that dissolve in water.
- Asteroids in Kuiper belt (richer in volatiles) are likely to have nitrogen in the form of Ammonia ice. However, the delta vee to move elements this deep into the sun's gravity well is huge (~23 km/s). However, a low cost method such as magnetic sails might work, given plenty of time.
- The water available on Mars in frozen form is already sufficient for a significative ocean.
- Drawing down the CO2 partial pressure is likely to be done by biological carbon sequestration. Carbon sequestration in a biosphere might store between 100 to 300 tons of carbon per hectare, or 10-30 kg/m2 (ref). Carbon sequestration would consume CO2 and free oxygen, increasing its concentration in the atmosphere. The carbon in the Martian atmosphere masses about 6.5e15 kg. This would be enough to supply 2 to 6e14 m2 of land with the required sequestrated carbon. As Mars' surface is only 1.44e14m2, the biosphere could absorb most, but not all, of the carbon. Additional CO2 would be stored in a Martian ocean, or as carbonates. Therefore excess CO2 is probably not a problem, the problem is adding the required buffer gas, most likely nitrogen. Some CO2 would need to remain in the atmosphere to run the CO2 cycle.
Note that covering the entire planet with soil, and then adding carbon to it is very slow. Fogg has suggested that growing trees, and moving the lumber outside the biosphere (say by burying it deep, or placing it above the atmosphere in the Olympus Mons caldera) would be a much faster way of drawing down CO2 levels, taking only thousands of years.
Long term prospects
The ultimate results of terraforming are disputed. Terraforming may have only a temporary effect, even if the effect lasts for some hundred or thousand years. Eventually, the solar wind may carry away most of the new atmosphere due to the insufficient magnetic fields of Mars, though this would take hundreds of millions of years. (See Atmospheric loss for more details.)
It has been suggested that the cost of terraforming a planet would be prohibitive. However, to a growing population on the surface of that planet it would most likely be considered a normal colonial function to ensure that daily colonial endeavours have a positive effect on the atmosphere. Also note that industry will naturally produce greenhouse and super greenhouse gases for 'free', so some terraforming is likely to happen just from having a human presence on Mars.
Artificial magnetic fields might also be created around Mars to reduce atmospheric losses to space. And the solar system contains sufficient resources to replenish Martian atmosphere indefinitely, but at a significant energy cost. Building space habitats might be a more practical long term objective for human occupation of space. Although space habitats may leak air faster than atmospheric loss from solar wind sputtering.
Partial terraforming
Parameter | Mars[4], mbar | Plants[5], mbar | Humans, mbar |
---|---|---|---|
Total pressure | 0.30-11.55 (6 average) | >10 | >250 |
Carbon dioxide (CO2) | 0.29-11.09 (5.76 average) | >0.15 | <0.5 |
Nitrogen (N2) | 0.01-0.22 (0.114 average) | >1-10 | - |
Oxygen (O2) | <0.015 | 1 | >130 |
Argon (Ar) | 0.09 | 0 | 0 |
Carbon Monoxide (CO) | 0.004 | 0 | 0 |
Water (H2O) | 0.0018 | ~10 | ~10 |
While full terraforming to make Mars atmosphere suitable for breathable condition for humans will take hundreds perhaps thousands of generations, transformations to the atmosphere suitable for plants could be faster. Current requirements for plants to grow on Mars are based on atmospheric pressure. Mars polar caps have enough CO2 to provide 100 mbar (10 kPa) additional atmospheric pressure to the existing 6 millibars. This would probably be enough to create sustainable growth condition for plants.
Note that even adding 10 mbar of pressure would make liquid water stable over much of the planet (if locally it was warm enough). This would allow bacteria and perhaps lichen to grow at times.
The first job will be to add bacteria. Many types can live at Mars' current pressures, but they need liquid water, and thus the temperature must be increased. A key goal is to warm the planet by about 20 degrees C. This will cause the poles to evaporate, releasing massive amounts of CO2, and a good deal of water. The increased pressure will result in liquid water appearing for short times (typically it will freeze at night). But even transient water is enough for bacteria to start spreading. See Bacteria Colonists for more information.
To add enough pressure to make using pressure suit unnecessary for humans, atmospheric pressure needs to rise to at least 100 mbar (10 kPa) or ~10% of Earth atmospheric pressure.
To make it so a breathing mask is not required, the pressure needs to be at least 250 mbar (25% Earth's pressure), AND the oxygen partial pressure must be increased, AND the carbon dioxide partial pressure must be reduced. Drawing down the CO2 partial pressure is likely to be done by biological carbon sequestration. Carbon sequestration in a biosphere might store between 100 to 300 tons of carbon per hectare, or 10-30 kg/m2 (ref). Carbon sequestration would consume CO2 and free oxygen, increasing its concentration in the atmosphere.
To get to a breathable atmosphere, a combination of oxygen, and various other gases must be generated. This might be composed of 118 mbar of nitrogen, 1 mbar of argon and neon, 1 mbar of water vapour and 130 mbar of oxygen (minimal requirement oxygen pressure). Note that the CO2 must be at trace levels for animals to breath. CO2 of 0.05% is the maximum we would want for a breathable atmosphere. (Argon and neon are not needed for life, but can act as a buffer gas.)
We would want to increase CO2 partial pressure to warm the planet, but would have to later draw down this CO2 level if we wish to make the planet habitable for animals.
Note that a partially terraformed world is still of use to humans. If we were to increase the pressure of the planet to 80 millibars, that is not enough for people to go on the surface with oxygen masks; they would need a pressure suit over most of the planet. But the LOWEST areas of the planet (e.g. the northern plains and Hellas Basin), would have high enough pressure to allow colonists to dispense with space suits and move about on the surface with simple gas masks. Further, the radiation from space, and solar UV would be reduced. (Not to Earth levels of safety, but better than current conditions.) Terraforming does not have to be 100% complete, to give safety and economic benefits to the habitants.
Paraterraforming is a subset of terraforming where terraforming takes place inside huge structures which cover some fraction of the planet's surface.
Ethics of Terraforming
This seems very straightforward to me (Rick). The universe is FILLED with sterile rocks. Life is rare and precious. Adding another world where life can live for millions or billions of years is a noble act of the highest order. Perhaps the 'purpose' of intelligent life is to spread life in all its forms across space.
Books on Terraforming
"Terraforming: Engineering Planetary Environments", by Martin J. Fogg, ISBN: 1-56091-609-5, is a highly detailed textbook on the subject. It is very hard to find. Perhaps the Mars Society could update it and republish it.
"New Earths: Restructuring Earth and Other Planets", by James Edward Oberg, ISBN: 0-8117-1007-6.
"Terraforming: The Creating of Habitable Worlds", by Martin Beech, ISBN: 978-0-387-09795-4.
References
- ↑ https://www.science.org/doi/10.1126/sciadv.adn4650%7CFeasiblity of Keeping Mars Warm With Nano-particles
- ↑ https://agupubs.onlinelibrary.wiley.com/doi/full/10.1002/2016GL072199 - Measurements of Nitrates and Perchlorates in Martian soil
- ↑ https://www.cell.com/the-innovation/fulltext/S2666-6758(24)00095-X
- ↑ Mahaffy, P. R.; Webster, C. R.; Atreya, S. K.; Franz, H.; Wong, M.; Conrad, P. G.; Harpold, D.; Jones, J. J.; Leshin, L. A.; Manning, H.; Owen, T.; Pepin, R. O.; Squyres, S.; Trainer, M.; Kemppinen, O.; Bridges, N.; Johnson, J. R.; Minitti, M.; Cremers, D.; Bell, J. F.; Edgar, L.; Farmer, J.; Godber, A.; Wadhwa, M.; Wellington, D.; McEwan, I.; Newman, C.; Richardson, M.; Charpentier, A. - Abundance and Isotopic Composition of Gases in the Martian Atmosphere from the Curiosity Rover, Nature 341, pp. 263-266. DOI:10.1126/science.1237966
- ↑ Christopher P. McKay, Owen B. Toon & James F. Kasting - Making Mars habitable, Nature 352, pp. 489-496. DOI:10.1038/352489a0
x- https://cca-reports.ca/wp-content/uploads/2021/03/Carbon-Sinks_EN_CH-3_Forests.pdf