Difference between revisions of "Cebrenia quadrangle"
Line 59: | Line 59: | ||
File:Hrad Vallis in Cebrenia2.jpg|Hrad Vallis may have been formed when the large Elysium Mons volcanic complex melted ground ice, as seen by THEMIS]]. | File:Hrad Vallis in Cebrenia2.jpg|Hrad Vallis may have been formed when the large Elysium Mons volcanic complex melted ground ice, as seen by THEMIS]]. | ||
− | File:Hrad | + | File:Hrad Vallisstreamlined.JPG|Streamlined islands in Hrad Vallis, as seen by HiRISE. |
</gallery> | </gallery> | ||
Revision as of 08:57, 7 January 2019

The Cebrenia quadrangle is one of a series of 30 quadrangle maps of Mars. The quadrangle covers from 120° to 180° east longitude (180° to 240° west longitude) and from 30° to 65° north latitude. It is also referred to as MC-7 (Mars Chart-7).[1] Parts of[Utopia Planitia and Arcadia Planitia lie in the Cebrenia quadrangle. [2] This article aims to describe the location and major features of this quadrangle. When you finish reading it, you will know what it looks like from orbit and from the surface. Some HiRISE images may even show how it looks from the altitude of an airplane or a helicopter.
Contents
- 1 Origin of name
- 2 Major Features
- 3 Hecates Tholus
- 4 Craters
- 5 Gullies
- 6 Pedestal craters
- 7 Channels
- 8 Volcano-ice interactions
- 9 Layered structures
- 10 Latitude dependent mantle
- 11 Glaciers
- 12 Lineated Valley Fill
- 13 Chaos
- 14 Ice exposed in new craters
- 15 Cones
- 16 Viking’s Findings
- 17 References
- 18 See Also
- 19 Recommended reading
- 20 External links
Origin of name
Cebrenia is a classical albedo feature on Mars. The feature is named after Cebrenia, a country near ancient Troy. The name was approved by the International Astronomical Union (IAU) in 1958.[3] [4]
Major Features
The major landscape features of the Cebrenia quadrangle are the large craters Mie and Stokes, a volcano called Hecates Tholus, and a group of mountains, named the Phlegra Montes. Most of the quadrangle is characterized as flat and smooth; hence, the rather large craters Mie and Stokes really stand out. The Galaxias Region is classified as an area of chaos; the ground just seems to have collapsed there. Some features found here are very common on Mars, but rare—if they exist at all—on the Earth. Pedestal craters, lineated valley fill (LVF), latitude dependent mantle (LDM), various-shaped layered features, and brain terrain are in this category. As on Earth, this region of Mars displays channels, layered terrain, cones, impact craters, gullies, and evidence of glaciers.[5] Viking 2 landed near MieCrater on September 3, 1976. Its landing coordinates were 48° N and 226° W.[6]
Hecates Tholus
Scientists believe that Hecates Tholus erupted explosively about 350 million years ago, which is a not that long ago for Mars. These eruptions made depressions on the sides of the volcano. And just five million years ago, glacial deposits formed inside these depressions. Some valleys on Hecates show a parallel drainage pattern.[7] Hecates Tholus is in the Elysium Volcanic Region; the two other volcanoes there are Elysium Mons and Albor Tholus.
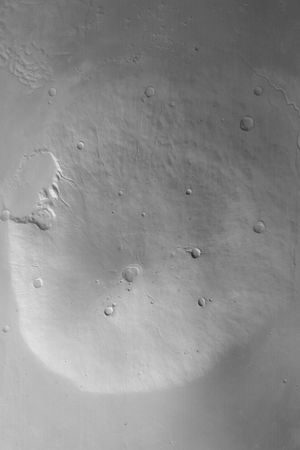
Craters
Impact craters generally have a rim with ejecta around them, in contrast volcanic craters usually do not have a rim or ejecta deposits.[8] Sometimes craters will display layers. Since the collision that produces a crater is like a powerful explosion, rocks from deep underground are tossed onto the surface. Consequently, craters can tell us what lies deep under the surface.
Mie Crater, as seen by CTX camera (on Mars Reconnaissance Orbiter). Viking II landed near Mie Crater in 1976.
Gullies
Pedestal craters
A pedestal crater is an impact crater which has its ejecta sitting above the surrounding terrain. This forms a raised platform (like a pedestal). These craters are produced when an impact crater ejects material that forms an erosion-resistant layer at the top, thus causing the immediate area to erode more slowly than the rest of the region. Some pedestals have been accurately measured to be hundreds of meters above the surrounding area. This means that hundreds of meters of material were eroded away. The result is that both the crater and its ejecta blanket stand above the surroundings. Pedestal craters were first observed during the Mariner missions.[9] [10] [11] [12] Much of the material under the pedestal crater’ ejecta may be ice. These types of craters may be useful for finding sources of water ice since they can be easily spotted and mapped from orbit.
Channels
There is enormous evidence that water once flowed in river valleys on Mars.[13] [14] Images of curved channels have been seen in images from Mars spacecraft dating back to the early seventies with the Mariner 9 orbiter.[15] [16] [17] [18] In fact, a study published in June 2017, estimated that the volume of water needed to carve all the channels on Mars was even larger than the proposed ocean that the planet may have had. Water was probably recycled many times from the ocean to rainfall around Mars.[19] [20]
Volcano-ice interactions
Large amounts of water ice are believed to be present just under the surface of Mars. Some channels lie near volcanic areas. When hot subsurface magma comes close to this ice, large amounts of liquid water and mud may be formed. Hrad Vallis in the Cebrenia quadrangle is close to the large volcano, Elysium Mons. It may have supplied heat to melt ice and make the channel. Hrad Vallis is pictured below.[21]
Layered structures
Some locations on Mars display layered features in craters, depressions, and next to mesas. These are probably the remains of materials that once covered a wide region. They may mostly consist of latitude dependent mantle which falls from the sky as ice-rich particles.
Latitude dependent mantle
Mantle may be composed of ice and dust that fell from the sky during past climatic conditions. Mantle could be a major source of water for future colonists because it is fairly common and could be mined for water by robotic machines.
Glaciers
Since the 60’s, as our spacecraft have studied Mars with more and more advanced cameras and other instruments, we have found more and more evidence for glaciers. [22] [23] [24] [25] [26] [27] [28] On Mars these glaciers are covered with rock and dust debris a few meters to a few tens of meters thick. Even though Mars today is too dry for any glaciers, this covering material has protected the underlying ice. [29] One would think that under today’s conditions any exposed ice would undergo sublimation and disappear into the thin Martian atmosphere. That is, it would go directly from a solid to a gas. However, the insulating effect of surface debris prevents the loss of ice.[26] [29] [30] [31]name="LevyBrainTerrain" />[32]
Lobate Debris Aprons
Features, called lobate debris aprons (LDA’s), around mesas are actually now accepted to be glaciers. These LDA’s looked like glaciers; final proof came with radar studies. The Shallow Subsurface Radar (SHARD) on board the Mars Reconnaissance Orbiter discovered that lobate debris aprons (LDA’s), around mesas were mostly ice under a thin rock debris cover. [33] [34] Ice was found both in the southern and northern hemispheres. [35] [36] [37]
Lineated Valley Fill
Another ice-rich feature is called lineated valley fill (LVF). [38] [39] [40] It covers many of the wide, flat valley floors of fretted terrain. Probably formed from the interaction of glaciers coming out of valleys and of mesas eroding, it has similar surface appearance of other supposed glaciers, including LDA. It looks like the human brain. Since the material covering ice is shaped into something resembling the human brain, it is named brain terrain. Brain terrain starts to form with cracks in the ice-rich surface. Sublimation along the cracks turns the cracks into small valleys. [41] [42] [43] [44] [45] [46] [47]
Chaos
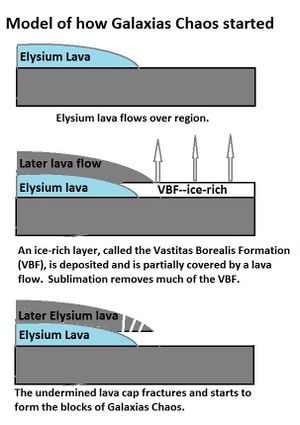
Some areas of Mars are classified as regions of “Chaos.” The ground seems to have collapsed. Often large blocks and hills are present in abundance. Many have large outflow channels associated with them. Galaxias Chaos in the Cebrenia quadrangle is different from most other chaotic regions. It does not have outflow channels, and it does not show a great elevation difference between it and the neighboring land area. Research by Pedersen and Head, published in 2010, suggests that Galaxias Chaos is the site of a volcanic flow that buried an ice-rich layer. That buried layer is a residue from water-rich materials deposited by large floods.[49] [50] Since the upper lava cap would not be supported evenly all over, it would crack. Along the cracks sublimation of water into the thin Martian atmosphere would be more intense. A blocky terrain that is typical of chaos would eventually develop. The sublimation process may have been facilitated by heat from magma movements. There are volcanoes, namely Elysium Montes and Hecates Tholus, close by which probably are surrounded by dikes, which would have heated the ground. Dikes occur when magma goes into cracks and weak areas rather than to the top of a volcano. They are widespread around most volcanoes. Ship rock, New Mexico contains typical dikes that have been exposed by erosion. Also, a warmer period in the past would have increased the amount of water sublimating from the ground.[51]
Ice exposed in new craters
Remarkable research, published in the journal Science in September 2009.[52] reported that some new craters on Mars show exposed, pure, water ice. Furthermore, scientists watched the ice disappear, presumably evaporating into the atmosphere by the process of sublimation. The ice is only a few feet deep. The Compact Imaging Spectrometer (CRISM)] on board the Mars Reconnaissance Orbiter (MRO ) confirmed that the bright substance was indeed water ice. The ice was found in a total of 5 locations. Three of the locations are in the Cebrenia quadrangle. These locations are 55.57 N 150.62 E (209.38 W), 43.28 N 176.9 E (183.1 W), and 45 N 164.5 E (195.5 W)g.[53] [54] [55] [56] This discovery proves that future colonists on Mars will be able to obtain water from a wide variety of locations. This ice can be dug up, melted, and then taken apart to provide fresh oxygen and hydrogen for rocket fuel. Hydrogen is the powerful fuel used by the space shuttle main engines. Robotic machines may be invented to mine and bring ice to future colonies.
Cones
Viking’s Findings
Viking 2’s lander at about 200 km west of Mie Crater in Utopia Planitia at 47.64 degrees N and 134.29 degrees E.[57] The location can easily be seen on maps of Mars because Mie Crater sits by itself. For over four years it imaged the surface, analyzed soil samples, and performed experiments that looked for life. The last message from Viking Lander 2 came to Earth on April 11,1980.[58] The last communication with the Viking 2 Orbiter occurred on July 25, 1978.[59] By the end of the Orbiter’s mission, it had returned nearly 16,000 images in its 706 orbits around the Red Planet.[60]
View of surface around Viking 2
Minerals detected in the soil were similar to those produced from the weathering of basaltic lavas on Earth. Dirt at this site contained abundant silicon and iron, in addition to significant amounts of magnesium, aluminum, sulfur, calcium, and titanium. Strontium and yttrium were found in trace amounts. Potassium was 5 times lower than the average in the Earth's crust. Sulfur and chlorine were similar to compounds that remain after sea water evaporates. Sulfur was more concentrated in the top crust of the soil than in the underlying bulk soil. Sulfur could be in the form of sulfates of sodium, magnesium, calcium, or iron. Iron sulfide is also possible. [61] Elements in the soil were found with an X-ray fluorescence spectrometer.[62] Mineral models suggest that the soil could be a mixture of about 90% iron-rich clay, about 10% magnesium sulfate (kieserite?), about 5% carbonate (calcite), and about 5% iron oxides (hematite, magnetite, goethite?). These minerals are typical weathering products of mafic igneous rocks. The dark, igneous rock basalt is believed to make up much of the surface of Mars, as it does for the Earth.[63] [64] [65] Studies with magnets aboard the Viking landers indicated that the soil is between 3 and 7 percent magnetic materials by weight. The magnetic chemicals could be magnetite and maghemite. These could come from the weathering of basalt rock.[66] [67]
Map showing locations of Viking landers and Pathfinder Note Viking 2 in far North.
Viking 2 gathered weather data for over 4 years while active on the surface. Temperatures at this location varied from a low of -189.4 degrees F (-123 C, 150 K) to a high of -9.4 degrees F (-23 C, 250 K). As these temperatures were recorded in the far north, cold temperatures would be expected. Furthermore, pressure changes, seasonal dust storms, , and transport of atmospheric gases between the polar caps were observed..[68] Three experiments on Viking looked for life. Granted that some activity was observed, most scientists now believe that the data were from inorganic chemical reactions of the soil. Nevertheless, a few scientists still to this day believe the results were due to living organisms.[69] [70] [71] Because no organic chemicals were found in the soil, most scientists concluded there was no life detected at this location. However, dry areas of Antarctica do not have detectable organic compounds either, but they have organisms living in the rocks.[72] Early on, some suggested that the lack of a protective ozone layer would cause UV light to sterilize the soil surface. Mars has almost no ozone layer, so UV light sanitizes the surface and may produce peroxides that would change any organic chemicals.[73] These theories did seem to be validated, decades later when the Phoenix Lander detected the chemical perchlorate in the Martian Soil. Perchlorate, a strong oxidant may have wiped out all traces of organic matter in the Martian soil.[74] Carbon-based life would be difficult at the soil surface, if perchlorates were pervasive on Mars. In addition, a study published in the Journal of Geophysical Research in 2010,suggested that organic compounds were actually present in the soil analyzed by both Viking 1 and 2. The study's authors discovered that perchlorate will destroy organics when heated and will produce chloromethane and dichloromethane--the same chlorine compounds discovered by both Viking landers when they performed the tests on Mars. [75] [76] [77] [78]Since perchlorate would have broken down any Martian organics, there could be life at the Viking sites.[79] [80]
References
- ↑ Davies, M.E.; Batson, R.M.; Wu, S.S.C. “Geodesy and Cartography” in Kieffer, H.H.; Jakosky, B.M.; Snyder, C.W.; Matthews, M.S., Eds. Mars. University of Arizona Press: Tucson, 1992.
- ↑ Davies, M.E.; Batson, R.M.; Wu, S.S.C. “Geodesy and Cartography” in Kieffer, H.H.; Jakosky, B.M.; Snyder, C.W.; Matthews, M.S., Eds. Mars. University of Arizona Press: Tucson, 1992.
- ↑ http://planetarynames.wr.usgs.gov/Feature/1095
- ↑ https://planetarynames.wr.usgs.gov/
- ↑ https://en.wikipedia.org/wiki/Cebrenia_quadrangle#Physiography_and_geology
- ↑ Ezell E. K., Ezell L. N. On Mars: Exploration of the Red Planet. 1958-1978. (Chapter 10). The NASA History Series. Scientific and Technical Information Branch, 1984. NASA, Washington, D.C.
- ↑ Hugh H. Kieffer (1992). Mars. University of Arizona Press. ISBN 978-0-8165-1257-7.
- ↑ Template:Cite book
- ↑ http://hirise.lpl.eduPSP_008508_1870
- ↑ Bleacher, J. and S. Sakimoto. Pedestal Craters, A Tool For Interpreting Geological Histories and Estimating Erosion Rates. LPSC
- ↑ http://themis.asu.edu/feature_utopiacraters
- ↑ McCauley, John F. (December 1972). "Mariner 9 Evidence for Wind Erosion in the Equatorial and Mid-Latitude Regions of Mars". Journal of Geophysical Research. 78 (20): 4123–4137(JGRHomepage).
- ↑ Baker, V., et al. 2015. Fluvial geomorphology on Earth-like planetary surfaces: a review. Geomorphology. 245, 149–182.
- ↑ Carr, M. 1996. in Water on Mars. Oxford Univ. Press.
- ↑ Baker, V. 1982. The Channels of Mars. Univ. of Tex. Press, Austin, TX
- ↑ Baker, V., R. Strom, R., V. Gulick, J. Kargel, G. Komatsu, V. Kale. 1991. Ancient oceans, ice sheets and the hydrological cycle on Mars. Nature 352, 589–594.
- ↑ Carr, M. 1979. Formation of Martian flood features by release of water from confined aquifers. J. Geophys. Res. 84, 2995–300.
- ↑ Komar, P. 1979. Comparisons of the hydraulics of water flows in Martian outflow channels with flows of similar scale on Earth. Icarus 37, 156–181.
- ↑ http://spaceref.com/mars/how-much-water-was-needed-to-carve-valleys-on-mars.html
- ↑ Luo, W., et al. 2017. New Martian valley network volume estimate consistent with ancient ocean and warm and wet climate. Nature Communications 8. Article number: 15766 (2017). doi:10.1038/ncomms15766
- ↑ http://themis.asu.edu/zoom-20020715a.html
- ↑ cite journal | last1 = Squyres | first1 = S.W. | last2 = Carr | first2 = M.H. | year = 1986 | title = Geomorphic evidence for the distribution of ground ice on Mars | url = | journal = Science | volume = 213 | issue = | pages = 249–253 | doi = 10.1126/science.231.4735.249 |
- ↑ cite journal | last1 = Head | first1 = J.W. | last2 = Marchant | first2 = D.R. | last3 = Dickson | first3 = J.L. | last4 = Kress | first4 = A.M. | year = 2010 | title = Criteria for the recognition of debris-covered glacier and valley glacier landsystem deposits | url = | journal = Earth Planet. Sci. Lett. | volume = 294 | issue = | pages = 306–320 | doi=10.1016/j.epsl.2009.06.041 |
- ↑ cite journal | last1 = Holt | first1 = J.W. | display-authors = 1 | last2 = et al | year = 2008 | title = Radar sounding evidence for buried glaciers in the southern mid-latitudes of Mars | url = | journal = Science | volume = 322 | issue = | pages = 1235–1238 | doi=10.1126/science.1164246 | pmid=19023078|
- ↑ | last1 = Morgan | first1 = G.A. | last2 = Head | first2 = J.W. | last3 = Marchant | first3 = D.R. | year = 2009 | title = Lineated valley fill (LVF) and lobate debris aprons (LDA) in the Deuteronilus Mensae northern dichotomy boundary region, Mars: Constraints on the extent, age and episodicity of Amazonian glacial events | url = | journal = Icarus | volume = 202 | issue = | pages = 22–38 | doi=10.1016/j.icarus.2009.02.017 |
- ↑ 26.0 26.1 cite journal | last1 = Plaut | first1 = J.J. | last2 = Safaeinili | first2 = A. | last3 = Holt | first3 = J.W. | last4 = Phillips | first4 = R.J. | last5 = Head | first5 = J.W. | last6 = Sue | first6 = R. | last7 = Putzig | first7 = A. | year = 2009 | title = Frigeri Radar evidence for ice in lobate debris aprons in the mid-northern latitudes of Mars | doi = 10.1029/2008gl036379 | journal = Geophys. Res. Lett. | volume = 36 | issue = | page = L02203 |
- ↑ cite journal | last1 = Baker | first1 = D.M.H. | last2 = Head | first2 = J.W. | last3 = Marchant | first3 = D.R. | year = 2010 | title = Flow patterns of lobate debris aprons and lineated valley fill north of Ismeniae Fossae, Mars: Evidence for extensive mid-latitude glaciation in the Late Amazonian | url = | journal = Icarus | volume = 207 | issue = | pages = 186–209 | doi=10.1016/j.icarus.2009.11.017 |
- ↑ cite journal | last1 = Arfstrom | first1 = J. | year = 2005 | title = Terrestrial analogs and interrelationships | url = | journal = Icarus | volume = 174 | issue = | pages = 321–335 | doi=10.1016/j.icarus.2004.05.026 | bibcode=2005Icar..174..321A
- ↑ 29.0 29.1 cite journal | last1 = Williams | first1 = K. E. | display-authors = 1 | last2 = et al | year = 2008 | title = Stability of mid-latitude snowpacks on Mars | url = | journal = Icarus | volume = 196 | issue = 2| pages = 565–577 | doi=10.1016/j.icarus.2008.03.017 |
- ↑ cite journal | doi = 10.1038/nature03359 | last1 = Head | first1 = J. | date = 2005 | last2 = Neukum | first2 = G. | last3 = Jaumann | first3 = R. | last4 = Hiesinger | first4 = H. | last5 = Hauber | first5 = E. | last6 = Carr | first6 = M. | last7 = Masson | first7 = P. | last8 = Foing | first8 = B. | last9 = Hoffmann | first9 = H. | last10 = Kreslavsky | first10 = M. | last11 = Werner | first11 = S. | last12 = Milkovich | first12 = S. | last13 = Van Gasselt | first13 = S. | last14 = Co-Investigator Team | first14 = The Hrsc | title = Tropical to mid-latitude snow and ice accumulation, flow and glaciation on Mars | url = | journal=Nature | volume = 434 | issue = 7031| pages = 346–350 | pmid=15772652|
- ↑ Head, J., et al. 2009. Northern mid-latitude glaciation in the Late Amazonian period of Mars: Criteria for the recognition of debris-covered glacier and valley glacier landsystem deposits. Earth and Planetary Science Letters. Doi:10.1016/j.epsl.2009.06.041
- ↑ Hubbard, Bryn, et al. "Geomorphological characterization and interpretation of a mid-latitude glacier-like form: Hellas Planitia, Mars." Icarus 211.1 (2011): 330-346.
- ↑ Plaut, J. et al. 2008. Radar Evidence for Ice in Lobate Debris Aprons in the Mid-Northern Latitudes of Mars. Lunar and Planetary Science XXXIX. 2290.pdf
- ↑ http://hirise.lpl.arizona.edu/PSP_009535_2240
- ↑ cite journal | last1 = Holt | first1 = J. | last2 = Safaeinili | first2 = A. | last3 = Plaut | first3 = J. | last4 = Head | first4 = J. | last5 = Phillips | first5 = R. | last6 = Seu | first6 = R. | last7 = Kempf | first7 = S. | last8 = Choudhary | first8 = P. | last9 = Young | first9 = D. | last10 = Putzig | first10 = N. | last11 = Biccari | first11 = D. | last12 = Gim | first12 = Y. | year = 2008 | title = Radar sounding evidence for buried glaciers in the southern mid-latitudes of Mars | url = | journal = Science | volume = 322| issue = | pages = 1235–1238| doi = 10.1126/science.1164246 | pmid=19023078|
- ↑ cite journal | last1 = Plaut | first1 = J. | last2 = Safaeinili | first2 = A. | last3 = Holt | first3 = J. | last4 = Phillips | first4 = R. | last5 = Head | first5 = J. | last6 = Seu | first6 = R. | last7 = Putzig | first7 = N. | last8 = Frigeri | first8 = A. | year = 2009 | title = Radar evidence for ice in lobate debris aprons in the midnorthern latitudes of Mars | url = | journal = Geophys. Res. Lett. | volume = 36| issue = | page = | doi = 10.1029/2008GL036379 |
- ↑ Holt, J., et al. 2008. Radar Sounding Evidence for Ice within Lobate Debris Aprons, near Hellas Basin, Mid-southern Latitudes of Mars. Lunar and Planetary Science XXXIX. 2441.pdf
- ↑ Carr, M. 2006. The Surface of Mars. Cambridge University Press. ISBN|978-0-521-87201-0
- ↑ Squyres, S. 1978. Martian fretted terrain: Flow of erosional debris. Icarus: 34. 600-613.
- ↑ Levy. et al. 2007. Lineated valley fill and lobate debris apron stratigraphy in Nilosyrtis Mensae, Mars: Evidence for phases of glacial modification of the dichotomy boundary. J. Geophys. Res. 112
- ↑ Mellon, M. 1997. Small-scale polygonal features on Mars: Seasonal thermal contraction cracks in permafrost. J. Geophysical Res: 102. 25,617-625,628.
- ↑ Levy, J. et al. 2009. Concentric crater fill in Utopia Planitia: History and interaction between glacial "brain terrain" and periglacial processes. Icarus: 202. 462-476.
- ↑ cite journal | author = Souness C., Hubbard B. | year = 2013 | title = An alternative interpretation of late Amazonian ice flow: Protonilus Mensae, Mars | url = | journal = Icarus | volume = 225 | issue = | pages = 495–505 | doi=10.1016/j.icarus.2013.03.030 |
- ↑ cite journal |author1=Head, J. |author2=D. Marchant |lastauthoramp=yes| date = 2006 | title = Modification of the walls of a Noachian crater in northern Arabia Terra (24E, 39N) during mid-latitude Amazonian glacial epochs on Mars: Nature and evolution of lobate debris aprons and their relationships to lineated valley fill and glacial systems | journal = Lunar Planet. Sci | volume = 37 | page = Abstract # 1126
- ↑ cite journal | author = Kress, A., J. Head | date = 2008 | title = Ring-mold craters in lineated valley fill and lobate debris aprons on Mars: Evidence for subsurface glacial ice | journal = Geophys. Res. Lett. | volume = 35 | page = L23206–8 | doi=10.1029/2008gl035501|
- ↑ cite journal | author = Baker, D. | date = 2010 | title = Flow patterns of lobate debris aprons and lineated valley fill north of Ismeniae Fossae, Mars: Evidence for extensive mid-latitude glaciation in the Late Amazonian | journal = Icarus | volume = 207 | pages = 186–209 | doi = 10.1016/j.icarus.2009.11.017 | last2 = Head | first2 = James W. | last3 = Marchant | first3 = David R.
- ↑ cite journal |author1=Kress., A. |author2=J. Head |lastauthoramp=yes| date = 2009 | title = Ring-mould craters on lineated valley fill, lobate debris aprons, and concentric crater fill on Mars: Implications for near-surface structure, composition, and age | journal = Lunar Planet. Sci | volume = 40 | page = abstract 1379
- ↑ Pedersen, G. and J. Head. 2011. Chaos formation by sublimation of volatile-rich substrate: evidence from Galaxias Chaos, Mars. Icarus. 211: 316-329.
- ↑ Kreslavsky, Mikhail A. (2002). "Fate of outflow channel effluents in the northern lowlands of Mars: The Vastitas Borealis Formation as a sublimation residue from frozen ponded bodies of water". Journal of Geophysical Research.
- ↑ Carr, Michael H. (2003). "Oceans on Mars: An assessment of the observational evidence and possible fate" (PDF). Journal of Geophysical Research. 108 (E5): 5042.
- ↑ Pedersen, G. and J. Head. 2011. Chaos formation by sublimation of volatile-rich substrate: evidence from Galaxias Chaos, Mars. Icarus. 211: 316-329.
- ↑ Byrne, S. et al. 2009. Distribution of Mid-Latitude Ground Ice on Mars from New Impact Craters: 329.1674-1676
- ↑ https://www.space.com/7333-water-ice-exposed-mars-craters.html
- ↑ Kossacki, K., N. Thomas. 2011. The evolution of exposed ice in a fresh mid-latitude crater on Mars. Icarus: 211, 195-206.
- ↑ https://science.nasa.gov/science-news/science-at-nasa/2009/24sep_martianice
- ↑ https://www.nasa.gov/jpl/mro/mars-reconnaissance-orbiter-pia17609.html#.XC-c6FxKjIU
- ↑ https://nssdc.gsfc.nasa.gov/nmc/spacecraft/display.action?id=1975-083C
- ↑ https://www.jpl.nasa.gov/news/fact_sheets/viking.pdf
- ↑ Kieffer, H., et al. (eds.) 1992. Mars. The University of Arizona Press. Tucson
- ↑ https://nssdc.gsfc.nasa.gov/nmc/spacecraft/display.action?id=1975-083A
- ↑ Clark, B. et al. 1976. Inorganic Analysis of Martian Samples at the Viking Landing Sites. Science: 194. 1283-1288.
- ↑ https://nssdc.gsfc.nasa.gov/nmc/spacecraft/display.action?id=1975-083C
- ↑ Baird, A. et al. 1976. Mineralogic and Petrologic Implications of Viking Geochemical Results From Mars: Interim Report. Science: 194. 1288-1293.
- ↑ Toulmin III, P. et al. 1977. Geochemical and Mineralogical Interpretation of the Viking Inorganic Chemical Results. Journal of Geophysical Research: 82. 4625-4634.
- ↑ Clark, B. et al. 1982. Chemical Composition of Martian Fines. Journal of Geophysical Research: 87. 10059-10097
- ↑ Hargraves, R. et al. 1976. Viking Magnetic Properties Investigation: Further Results. Science: 194. 1303-1309.
- ↑ Arvidson, R, A. Binder, and K. Jones. The Surface of Mars. Scientific American
- ↑ https://nssdc.gsfc.nasa.gov/planetary/viking.html
- ↑ https://news.nationalgeographic.com/news/2012/04/120413-nasa-viking-program-mars-life-space-science/
- ↑ http://edition.cnn.com/2000/TECH/space/11/07/mars.sample/
- ↑ https://www.youtube.com/watch?v=MX7vRut_lUg
- ↑ Friedmann, E. 1982. Endolithic Microorganisms in the Antarctic Cold Desert. Science: 215. 1045-1052.
- ↑ Hartmann, W. 2003. A Traveler's Guide to Mars. Workman Publishing. NY NY.
- ↑ Alien Rumors Quelled as NASA Announces Phoenix Perchlorate Discovery. A.J.S. Rayl, August 6, 2008.
- ↑ Navarro-González, Rafael; et al. (2011). "Comment on "Reanalysis of the Viking results suggests perchlorate and organics at midlatitudes on Mars". Journal of Geophysical Research. 116 (E12): E12001.
- ↑ Navarro–Gonzáles, Rafael; Vargas, Edgar; de la Rosa, José; Raga, Alejandro C.; McKay, Christopher P. (2010-12-15). "Reanalysis of the Viking results suggests perchlorate and organics at midlatitudes on Mars". Journal of Geophysical Research: Planets. 115 (E12010): E12010.
- ↑ Navarro-González, Rafael; Vargas, Edgar; de la Rosa, José; Raga, Alejandro C.; McKay, Christopher P. (2011). "Correction to "Reanalysis of the Viking results suggests perchlorate and organics at midlatitudes on Mars"". Journal of Geophysical Research. 116 (E8): E08011.
- ↑ Navarro-González, Rafael; Navarro, Karina F.; de la Rosa, José; Iñiguez, Enrique; Molina, Paola; Miranda, Luis D.; Morales, Pedro; Cienfuegos, Edith; Coll, Patrice; et al. (2006). "The limitations on organic detection in Mars-like soils by thermal volatilization-gas chromatography-MS and their implications for the Viking results". Proceedings of the National Academy of Sciences. 103 (44): 16089–94.
- ↑ https://www.sciencedaily.com/releases/2010/09/100904081050.htm
- ↑ https://web.archive.org/web/20140819090841/http://www.gillevin.com/Mars/Levin-Straat_Mars_Society_Paper_8-8-14.pdf
See Also
- Geography of Mars
- Glaciers on Mars
- Hecates Tholus
- High Resolution Imaging Science Experiment (HiRISE)
- Layers on Mars
- Martian features that are signs of water ice
- Martian gullies
- Rivers on Mars
- Sublimation
- Sublimation landscapes on Mars
- Viking 2
Recommended reading
- Grotzinger, J., R. Milliken (eds.). 2012. Sedimentary Geology of Mars. Tulsa: Society for Sedimentary Geology.
- Kieffer, H., et al. (eds) 1992. Mars. The University of Arizona Press. Tucson
- September 30, 1977 issue of the Journal of Geophysical Research, "Scientific Results of the Viking Project", vol. 82, no. 28.
- history.nasa.gov/SP-4212/ch11
External links
- https://www.youtube.com/watch?v=TG5X37q-j08 Viking Mars Landers: "19 Minutes to Earth" 1978 NASA; Mars Science Data Summary
- https://www.youtube.com/watch?v=JR9HvTPP-W0 Landing On Mars Viking's Historic Mission (1987)
- https://www.youtube.com/watch?v=nZc62KaN980 Viking-2 on Mars (1976)
- https://www.youtube.com/watch?v=MX7vRut_lUg Was Microbial Life on Mars Discovered in 1976?